應用於多用戶編碼協力式通訊之高頻譜效率空時協定
全文
(2) 應用於多用戶編碼協力式通訊之高頻譜效率空時協定. Spectrally Efficient Space-Time Protocols for Multi-user Coded Cooperative Communications 研 究 生:王雋甫. Student: Chun-Fu Wang. 指導教授:李大嵩 博士. Advisor: Dr. Ta-Sung Lee. 吳卓諭 博士. Dr. Jwo-Yuh Wu. 國立交通大學 電信工程學系碩士班 碩士論文 A Thesis Submitted to Department of Communication Engineering College of Electrical and Computer Engineering National Chiao Tung University In Partial Fulfillment of the Requirements For the Degree of Master of Science In Communication Engineering June 2008 Hsinchu, Taiwan, Republic of China. 中 華 民 國 九 十 七 年 六 月.
(3) 應用於多用戶編碼協力式通訊之 高頻譜效率空時協定 學生:王雋甫. 指導教授:李大嵩 博士 吳卓諭 博士 Chinese Abstract. 國立交通大學電信工程學系碩士班. 摘要 在協力式通訊系統中,給中繼站使用的額外通道所造成的頻譜效率損失,是 其主要的缺點。近年來,有一種高頻譜效率的解決方案被提出來,稱作「編碼協 力式通訊」。在本論文中,吾人的目標為設計基於編碼協力式通訊的空時協定, 藉此達到更高的系統可靠度。吾人將提出兩種不同的協定: 空時(ST)及碼分配 式(CP)編碼協力式通訊,以上兩種協定皆能達到比傳統編碼協力式通訊更好 的表現,同時還能維持相同的傳輸速率以及功耗。透過成偶比對錯誤率的推導, 吾人證明這兩種通訊協定皆能達到最大多樣增益。此外,針對用戶之間通道不良 的情況,吾人也提出了穩健的方法及其分析。值得一提的是,雖然本論文僅對於 雙用戶及四用戶的情況做介紹,吾人很容易可將之延伸到其它用戶數量。此外, 協力式編碼的架構以及空時碼的選擇,也可以彈性地針對不同的需求來修改。. I.
(4) Spectrally Efficient Space-Time Protocols for Multi-user Coded Cooperative Communications English Abstract Student: Chun-Fu Wang. Advisor: Dr. Ta-Sung Lee Dr. Jwo-Yuh Wu. Department of Communication Engineering National Chiao Tung University. Abstract A major drawback of cooperative communication is the spectral efficiency loss due to additional channel needs for the relay. Recently, coded cooperation had been proposed as a spectrally efficient solution for user cooperation. In this thesis, our aim is to design space-time protocols under coded cooperation that achieve higher system reliability. We introduce two modified protocols: space-time (ST) coded cooperation and code partitioning (CP) coded cooperation. These protocols achieve remarkable gains comparing to the performance of conventional coded cooperation while maintaining the same data rate and transmit power. We conduct performance analysis in terms of pairwise error probabilities, showing that both protocols achieve extra diversity gain guaranteed by the space-time code used. Robust algorithms in case of poor inter-user link are also developed and analyzed. Although we demonstrate the case of two and four users, extension to other number of users is straightforward. Different types of code structures for cooperation and space-time coding are also flexible to choose depending on various needs. II.
(5) Acknowledgement I would like to express my deepest gratitude to my advisor, Dr. Ta-Sung Lee, for his enthusiastic guidance and great patience, especially in the training of oral presentation. I learned a lot from his positive attitude in many areas. Thanks are also offered to all members in the Communication System Design and Signal Processing (CSDSP) Lab. At last but not least, I would like to show my sincere thanks to my family for their invaluable love and support.. III.
(6) Contents Chinese Abstract ..........................................................................I English Abstract......................................................................... II Acknowledgement .................................................................... III Contents .................................................................................... IV List of Figures........................................................................... VI Acronym Glossary ................................................................. VIII Notations................................................................................... IX Chapter 1 Introduction................................................................ 1 Chapter 2 System model and Problem Formulation ................... 5 2.1 Review of Cooperation Network .....................................................................5 2.2 Review of Coded Cooperation.......................................................................10 2.2.1 Code Selection .................................................................................... 11 2.2.2 Transmission Model............................................................................12 2.3 Proposed Protocols Based on Coded Cooperation.........................................13 2.4 Summary ........................................................................................................14. Chapter 3 Spectrally Efficient Multi-user Coded Cooperation Using Space-Time Code ........................................................... 16 IV.
(7) 3.1 Protocols of ST-Coded Cooperation ..............................................................16 3.1.1 Code Structure and System Model......................................................17 3.1.2 Case of Data Exchange Failure...........................................................20 3.2 Performance Bounds of ST-Coded Cooperation............................................20 3.2.1 Pairwise Error Probability...................................................................21 3.2.2 Bit and Block Error Rate.....................................................................25 3.2.3 Impact of Data Exchange Failure .......................................................28 3.3 Computer Simulations ...................................................................................30 3.4 Summary ........................................................................................................34. Chapter 4 Spectrally Efficient Multi-user Coded Cooperation using Code Partitioning ............................................................ 36 4.1 Protocols of CP-Coded Cooperation..............................................................36 4.1.1 Code Structure and System Model......................................................37 4.1.2 Case of Information Exchange Failure ...............................................41 4.2 Performance Bounds of CP-Coded Cooperation ...........................................43 4.2.1 Impact of Data Exchange Failure .......................................................46 4.3 Computer Simulations...................................................................................48 4.4 Summary ........................................................................................................53. Chapter 5 Conclusions and Future Works................................. 55 Bibliography ............................................................................. 59. V.
(8) List of Figures Fig. 1-1. Cooperation between mobile users ...............................................................2 Fig. 1-2. System design of (a) Network coding [13], (b) Network coding [14], (c) Spatial reuse [15], (d) Coded cooperation[16].......................................................3 Fig. 2-1. Protocol of two-user cooperation..................................................................6 Fig. 2-2. Channel use in half-duplex two-user cooperation (TDMA) .........................7 Fig. 2-3. Channel use in half-duplex four-user cooperation (TDMA).........................9 Fig. 2-4. Structure of codeword in coded cooperation ..............................................12 Fig. 2-5. Channel use of coded cooperation with two users (TDMA) ......................12 Fig. 2-6. Comparison of channel uses for cooperation protocols (TDMA)...............15 Fig. 3-1. Channel use of ST-coded cooperation with two users (TDMA).................17 Fig. 3-2. Channel use of ST-coded cooperation with four users (TDMA) ................19 Fig. 3-3. Simulations and bounds of frame error rate (FER) in ST-coded cooperation. Equal uplink SNR, base code [15 17 13 15]........................................................32 Fig. 3-4. Frame error rate (FER) with imperfect inter-user channels. Equal uplink SNR, generator [15 17 13 15], inter-user FER=0.1 .............................................33 Fig. 3-5. Frame error rate (FER) with imperfect inter-user channels. Equal uplink SNR, generator [15 17 13 15], inter-user FER=0.1, adaptive algorithm .............34 Fig. 4-1. Channel use of CP-coded cooperation with two users (TDMA) ................38 Fig. 4-2. Structure of codeword in CP-coded cooperation (2-user case)...................38 Fig. 4-3. Channel use of CP-coded cooperation with four users (TDMA)................40 Fig. 4-4. Structure of codeword in CP-coded cooperation (4-user case)...................40 Fig. 4-5. Channel use of 4-user CP-coded cooperation with bad user1 – user2 link, VI.
(9) method 1...............................................................................................................42 Fig. 4-6. Channel use of 4-user CP-coded cooperation with bad user1 – user2 link, method 2...............................................................................................................43 Fig. 4-7. Simulations and bounds of frame error rate (FER) in CP-coded cooperation. Equal uplink SNR, base code [15 17 13 15 13 17]..............................................49 Fig. 4-8. Simulations and bounds of frame error rate (FER) in CP-coded cooperation. Equal uplink SNR, base code [15 17 13 15]........................................................50 Fig. 4-9. Frame error rate (FER) with imperfect inter-user channels. Equal uplink SNR, generator [15 17 13 15 13 17], inter-user FER=0.1, method 1 ..................52 Fig. 4-10. Frame error rate (FER) with imperfect inter-user channels. Equal uplink SNR, generator [15 17 13 15 13 17], inter-user FER=0.1, method 2 ..................53. VII.
(10) Acronym Glossary AF. amplify-and-forward. AWGN. additive white Gaussian noise. BER. bit error rate. BPSK. binary phase shift keying. CP. code partitioning. CRC. cyclic redundancy check. DF. decode-and-forward. FER. frame error rate. MGF. moment generating function. MIMO. multiple-input multiple-output. MISO. multiple-input single-output. ML. maximum likelihood. OSTBC. orthogonal space-time block code. PDF. probability density function. RCPC. rate-compatible punctured convolutional. SNR. signal-to-noise ratio. ST. space-time. STBC. space-time block code. STC. space-time coding. STTD. space-time transmit diversity. TDMA. time division multiple access. VIII.
(11) Notations ( ⋅). T. ( ⋅). H. transpose operator Hermitian operator. E {} ⋅. expectation operator. ⋅. Euclidean norm operator. ( ⋅). h. statistical averaging over a random variable. Es. transmit energy per bit. K. data block length. N. frame length. N0. noise variance. R. code rate. Uu. uth user. c. transmit codeword. h. channel coefficients. n. noise vector. r. received signal. s. transmit signal. z. detected signal. IX.
(12) Chapter 1 Introduction Various techniques are being investigated for meeting the goals of next generation wireless communications. Among these techniques, diversity is of great interests. In a multi-antenna communication system, one way to achieve diversity is to apply space-time coding (STC). For example, space–time trellis coding (STTC) is proposed in [1], which combines signal processing at the receiver with coding techniques for multiple transmit antennas. Space–time block coding (STBC), first discovered by Alamouti [2] and generalized in [3] and [4] to an arbitrary number of transmit antennas, is able to achieve the full diversity promised by the transmit and receive antennas. However, mobile devices may not be able to support multiple antennas due to size or other hardware constraints. In this case, since most wireless communication systems are operated in a multi-user scheme, the idea of user cooperation was born [5] [6]. The basic concept is shown in Fig. 1-1, in which every user has its partners. The users are responsible for transmitting not only their own data, but also the data of their partners they received and detected [7]. Diversity can be achieved since each mobile user sees an independent channel to the base station.. 1.
(13) Fig. 1-1.. Cooperation between mobile users. This form of space diversity is referred to as cooperative diversity in [8] (cf. user cooperation diversity of [6]). Space-time codes can be applied to the “virtual array” formed by the users. Anghel et al. extended the cooperative system by implementing a distributed Alamouti space-time code based on the multi-user scheme [9]. It is shown in [10] that cooperative diversity with appropriately designed codes realizes full spatial diversity gain. Common information at distributed points is required for applying distributed space-time codes. In other words, users have to exchange their data before cooperating. However, half-duplex systems which are commonly used in real world devices need additional channel for the transmission from user to partners, thus suffering from the loss in spectral efficiency [11][12]. Many solutions are proposed to reduce the above spectral loss (Fig. 1-2). The authors of [13] propose a network coding scenario for communication between two users. In [14] it is extended to the case of two users transmitting to one destination, 2.
(14) where multiple-access relay channel is used. In [15], the authors suggest spatially reusing the relay time slot. Although it causes interference between the users, this protocol has a spectral efficiency of K / ( K + 1) and is close to 1 when K is large. In the above cooperative models, the partner only repeats what it received. Recently, a different framework called coded cooperation was proposed ([16][17] and [18]), where signals are not repeated by the partner. They assume that the user data is protected by channel coding (which is commonly used in wireless communications) and partition the codeword into two sets: one set is transmitted by its own user and the other is transmitted by the partner. A sketch of system design of the mentioned cooperative models is given in Fig. 1-2.. (a). (b). (c). (d). Fig. 1-2. System design of (a) Network coding [13], (b) Network coding [14], (c) Spatial reuse [15], (d) Coded cooperation[16]. 3.
(15) Since there is no repetition of the codeword, the spectral efficiency of coded cooperation is 1. The works in [16]-[18] prove that it can achieve full diversity guaranteed by the number of users. Space-time coding can also be applied to coded cooperation. In [16], the authors suggest the use of space-time code which allows the users to capture better space-time diversity under fast fading. In [19], space-time overlay coding is used to achieve higher diversity gain when the partner nodes and their destination have multiple antennas. In this thesis, our main goal is to design and analyze the space-time coded cooperation protocols for single-antenna terminals under slow fading environments. It is intuitive to combine coded cooperation with distributed space-time code since it needs only half the time to complete the “data exchange” process comparing to conventional cooperation. In particular, we propose two modified protocols that achieve higher diversity order than conventional coded cooperation without the need for more antennas or channel resource. The remainder of the thesis is organized as follows. We give a brief review of the basic model of conventional cooperative scheme and coded cooperative scheme in Chapter 2, where concept of the proposed protocol will also be given. The first protocol will be described in Chapter 3. Bit error rate (BER) and frame error rate (FER) will be analyzed and given along with simulation results. Also, algorithms addressing the cases of data exchange failure will be considered. The second protocol will be described in Chapter 4. This new scheme is developed based on the discovery in Chapter 3. Performance analysis and simulation results will be given, and pros and cons of these two protocols will be discussed. Finally, we summarize the contributions of our works and give an outline of possible extended research in Chapter 5.. 4.
(16) Chapter 2 System model and Problem Formulation In this Chapter we review the concept and model of conventional wireless cooperative communication and coded cooperative communication. We introduce the problem of loss in spectral efficiency due to the additional channel uses in half-duplex systems under conventional cooperative scheme. Then, a different scheme called coded cooperation which compensates the spectral efficiency loss will be given. Finally, we introduce the proposed cooperation scheme to further utilize the benefits in cooperation, thus achieves higher transmission reliability. For the model of the cooperative system throughout this thesis, a narrow-band transmission in frequency-flat and slow fading channel with additive noise is considered to isolate the benefits of cooperation diversity. Extension to frequency- and time-selective fading channel can be naturally done, but the cooperation gain will not be substantial since other kind of diversity can be exploited in that system.. 2.1 Review of Cooperation Network Consider a system in Fig. 2-1 which two users U1 ,U 2 (ex. mobile phones) are 5.
(17) communicating with a destination D (base station). We assume all terminals are equipped with single antenna.. Step 1. Step 2. U1. U1. s1. s1. s2. D. U2. D s2. U2. Step 3. Step 4 U1. U1. s 2. D. D. s1. U2 Fig. 2-1.. U2 Protocol of two-user cooperation. We define step 1, 2 as “broadcast phase”, where two users separately transmit their information to the destination and the other user. We call it broadcast phase because it utilizes the broadcast nature of wireless channel. Step 3, 4 are defined as “relay phase”, where the two user act as a “relay” of each other, passing the information received from other user to the destination. Throughout the 4-step transmission, two copies of one user data will be transmitted by different user in broadcast phase and relay phase, separately. There are commonly two relaying protocols:. amplify-and-forward. (AF). and. decode-and-forward. (DF).. In. amplify-and-forward mode, relays amplify their received signals subject to their own power constraint. In decode-and-forward mode, relays fully decode the information and retransmit it to the destination. Both of these protocols achieve full diversity (of order 2 under 2-user case) in appropriate conditions. [20] 6.
(18) In these cooperation protocols, relays have to do both receiving and transmitting. To preserve time for the relaying process, a straightforward way is to make relays receiving and transmitting at the same time. However, implementation of this kind of full-duplex system (transmitting and receiving at the same time in the same frequency band) is less practicable due to the limitation of current radio hardware. Instead, half-duplex operation is commonly used in such cooperation protocols. That means broadcast and relay phase need to be separated into different channel uses. An example is shown in Fig. 2-2. Assuming time division multiple access (TDMA) is used, a total of 4 time slots are needed for each user to transmit one data.. user1 data. (antenna). user2 data user1 user2 time. . broadcast phase relay phase Fig. 2-2. Channel use in half-duplex two-user cooperation (TDMA) Define the channel coefficients between user U u and destination as hu , where u = 1, 2 . Assume all channels are independent, flat fading and quasi-static over one phase. Assuming binary phase-shift keying (BPSK) modulation is used, we define the baseband-equivalent su = ⎡⎣ su ,1. discrete-time. symbols. transmitted. by. user. Uu. as. su ,2 " su , N ⎤⎦ , where su ,i ∈ {−1, +1}, u = {1, 2}, i = 1," , N and N is the T. frame length. The signal received by destination is ru = hu Es su + nu. 7. (2.1).
(19) where Es is the transmit energy per bit, hu is the fading coefficient between user U u and destination with hu ~ Rayleigh (σ ) , hu = X + jY and X , Y ~ N ( 0, σ 2 ) . nu is N0 2. a vector of additive white Gaussian noise (AGWN) with zero mean and variance per dimension.. Now we denote ru( b ) as the received signal for user U u data at destination in broadcast phase. In the same way, ru( r ) denotes the received signal for user U u data at destination in relay phase. Thus the received signal at the destination in the four time slots can be written as ⎧⎪r2( r ) = h1( r ) Es s 2 + n(2r ) ⎨ (r ) (r) (r ) ⎪⎩r1 = h2 Es s1 + n1. ⎧⎪r1( b ) = h1( b ) Es s1 + n1( b ) , ⎨ (b) (b) (b) ⎪⎩r2 = h2 Es s 2 + n 2. Note that the channel coefficients are added with superscripts. (2.2). (b). and. (r ). to. distinguish the two phases. They are also added to the noise vector. The transmitted symbols at the relays s1 , s 2 are added with tilde because they are not always equal to the transmitted symbols at the source. In AF mode, s1 , s 2 are normalized signal received from the source, that is ⎧ r1,2 h E s + n1,2 = 1,2 s 1 ⎪s1 = 2 2 ⎪ Es h1,2 + N1,2 E r1,2 ⎪ ⎨ h2,1 Es s2 + n 2,1 r2,1 ⎪ s = 2 = ⎪ 2 2 Es h2,1 + N 2,1 E r2,1 ⎪ ⎩. { }. (2.3). { }. Note that r1,2 and r2,1 denote the received signals at user U 2 and U1 , respectively. These can be written in the form of ⎧⎪r1,2 = h1,2 Es s1 + n1,2 ⎨ ⎪⎩r2,1 = h2,1 Es s2 + n 2,1. 8. (2.4).
(20) where h1,2 , h2,1 denote the channel coefficients from user U1 to U 2 and from U 2 to U1 , respectively. n1,2 , n 2,1 denote the noise received by the relay, and N1,2 , N 2,1 is the noise power. In DF mode, the relays and the source will transmit the same information if the relays decode what they received correctly, that is, s1 = s1 , s 2 = s2 .. Half-duplex cooperation protocols suffer from the loss in spectral efficiency due to the two channel uses required for the transmission from the source to the relay and relay to destination, which causes a pre-log factor 1/2 in the corresponding rate expressions [11]. The protocol can be easily extended to more user cases, for example, Fig. 2-3 demonstrates a 4-user scheme. A diversity order of 4 can be achieved for each user’s data, but note that the length of the relay phase is three times longer than in 2-user case. Thus the loss of spectral efficiency is even severer. user1 data. user3 data. user2 data. user4 data. (antenna). user1 user2 user3 user4. time. relay phase broadcast phase Fig. 2-3. Channel use in half-duplex four-user cooperation (TDMA). 9.
(21) 2.2 Review of Coded Cooperation One way to avoid the additional channel use is using coded cooperation ([16][17] and [18]). Coded cooperation combines the idea of user cooperation with channel coding, which is commonly used in wireless communication systems. Assume data of all users are protected by channel coding. We call the channel code used as base code. Apart from the conventional cooperation protocol mentioned in Section 2.1 where relays repeat the received data, relays in coded cooperation decode and re-encode the received data using different encoder, then transmit it to the destination. More specifically, the base code is partitioned into two sub-codes: one is transmitted by the source and the other is transmitted by the relay, we call them broadcast sub-code and. relay sub-code, respectively. The basic idea is that the data exchanged between users is encoded by a shorter sub-code (broadcast sub-code) to preserve channel resource. Relay decodes the received data and encodes it into another sub-codeword (relay sub-code), then transmits it to the destination. The destination combines the codewords received from source and rebuilds the original base codeword for decoding. It is important to note that relay needs to successfully decode the data received from the source to do cooperation. The data is now only protected by shorter codes, but it is tolerable since the channel between users is usually better than the channel to the destination (considering cellular phone system for instance, the distance to nearby mobile user are much shorter than the distance to the base station) Consider 2-user case. For a rate-R base code, assuming there are K data bits per block, we have N =. K coded bits. The N-bits codeword is divided into two R. sub-codewords (for broadcast and relay phase, respectively). The length of each 10.
(22) b r sub-code needs not to be the same. We define N ( ) , N ( ) as the lengths of the. sub-codes for broadcast and relay phase, respectively. Note that N ( b ) + N ( r ) = N . We focus on TDMA scheme which is similar to the scheme in Section 2.1. In the broadcast phase, each user transmits its broadcast sub-codeword. If the relay successfully decodes the source data, it re-encodes the data using relay sub-code and transmits. Since the total transmitted bits of a data block is still N, no more channel resource is needed compared to direct transmission scheme, that is, no spectral efficiency loss.. 2.2.1 Code Selection Various channel coding methods can be used in the coded cooperation protocols. For example, the base code may be a block or convolutional code or a combination of both [16]. Partitioning of the codeword can be achieved by puncturing, product codes or other forms of concatenation. Convolutional codes with puncturing are used in [16]~[18], but with different generators: [16] uses a rate-compatible punctured convolutional (RCPC) code proposed in [21], where [18] uses a self-designed code for specific purpose. For the models in [18], the authors use convolutional codes with rate-1/4. The generator is [15 17 13 15], the codeword length is 260 bits and the puncturing patterns b r are [1 1 0 0] and [0 0 1 1]. Thus we have N ( ) = N ( ) = 130 and R ( ) = R ( ) =. b. structure of the codeword is given below:. 11. r. 1 . The 2.
(23) 1 code (260 coded bits) 4. apply puncturing pattern. 1 sub-codes 2 (130 coded bits each) two. . relay codeword braodcast codeword. Fig. 2-4. Structure of codeword in coded cooperation. 2.2.2 Transmission Model Based on the code structure given above, we denote the broadcast and relay sub-codewords of user U1 as c1( b ) , c1( r ) , respectively. In the same way, c(2b ) , c(2r ) denote the sub-codewords for user U 2 . The transmission model is similar to section 2.1, but different codewords are transmitted from the source and the relay. (Fig. 2-5) user1. user2. broadcast sub-codeword. (antenna). relay sub-codeword user1 user2 time. . broadcast phase relay phase Fig. 2-5. Channel use of coded cooperation with two users (TDMA) 12.
(24) Thus the received signal at the destination can be modeled as ⎧⎪r1( b ) = h1( b ) Es c1( b ) + n1( b ) , ⎨ (b) (b) (b) (b) ⎪⎩r2 = h2 Es c 2 + n 2. ⎧⎪r2( r ) = h1( r ) Es c(2r ) + n(2r ) ⎨ (r) (r) (r) (r ) ⎪⎩r1 = h2 Es c1 + n1. (2.5). Note that only DF mode can be used in coded cooperation, since the relay has to decode the received codeword in order to re-encode it into another codeword. The model can be extended to more user cases. However, it has similar problem to conventional cooperation. Longer relay phase is needed for all users to transmit the relay sub-codeword. It decreases the spectral efficiency and makes coded cooperation less attractive.. 2.3 Proposed Protocols Based on Coded Cooperation Considering the transmission model of coded cooperation (Fig. 2-5), there are several empty slots in both broadcast phase and relay phase. In broadcast phase, the transmission of different user’s data cannot be overlapped. One reason is that the destination is equipped with only one antenna and is unable to separate the signals of different users if they overlapped; the other reason is that a user has to listen to other user data in order to perform relaying. However, in relay phase, all users already know each other’s data (if decoded successfully). Thus some changes can be done here in order to improve the performance. We will introduce two modifications of the coded cooperation protocols, which we called space-time (ST) coded cooperation and code partition (CP) coded. cooperation. Detailed descriptions, simulations and the performance analyses of the protocols will be given in Chapter 3 and Chapter 4 for ST-coded and CP-coded 13.
(25) cooperation, respectively.. 2.4 Summary A comparison of all transmission protocols mentioned in this chapter is shown in Fig. 2-6 to make the difference of spectral efficiency more clear. The spectral efficiency loss in conventional 2-user cooperation protocol is clear shown in the figure. It uses twice more time slots compared to other protocols. Coded cooperation uses just half of the time but still achieves same diversity as conventional cooperation. For the proposed modification of coded cooperation protocols, we aim at the relay phase to achieve higher transmission reliability. In this chapter we review the system model of conventional cooperation and coded cooperation under multi-user schemes. It is shown that by using coded cooperation with appropriate choice of codes, we can avoid the loss of spectral efficiency while still achieving full diversity. Then we point out the potential of extracting more benefits from coded cooperation by using more reliable transmission in relay phase. Two modified protocols which can acquire higher diversity order (>2) without losing spectral efficiency are introduced.. 14.
(26) user1. user2. user3. user4. base codeword broadcast sub-codeword. relay sub-codeword. (Protocols). direct transmission. 2-user cooperation 2-user coded cooperation Proposed modification of 2-user coded cooperation. ?. ? time. Fig. 2-6. Comparison of channel uses for cooperation protocols (TDMA). 15.
(27) Chapter 3 Spectrally Efficient Multi-user Coded Cooperation Using Space-Time Code In this Chapter we introduce the first modification of coded cooperation: space-time (ST) coded cooperation. A detailed description of how this protocol works will be given. Performance bounds will be analyzed and showed along with simulation results. Cases when a user does not successfully decode the data from other users are also considered. We focus on 2-user coded cooperation with Alamouti space-time code for simplicity, but extension to more user cases with other types of space-time codes is straightforward.. 3.1 Protocols of ST-Coded Cooperation In coded cooperation, since all users know each other’s data after the broadcast phase, we can treat the users as “virtual antennas”. Since that, applying space-time code in relay phase to achieve transmit diversity is possible. In the following Sections we’ll give an example that shows how 2-user ST-coded cooperation works.. 16.
(28) 3.1.1 Code Structure and System Model Since there are two users in the cooperation scheme, we can apply Alamouti code [2] in the transmission of relay phase, the protocol is shown in Fig. 3-1. user1. user2. broadcast sub-codeword. (antenna). relay sub-codeword user1 user2 time. . broadcast phase relay phase Fig. 3-1. Channel use of ST-coded cooperation with two users (TDMA) Assume the same quasi-static fading channel as in Chapter 2, that is, the fading coefficient remains constant during the transmission of the space-time code (one phase). A diversity gain of 2 is extracted by the use of Alamouti code in the relay phase, thus we can expect a total diversity order of 3 for each user data since the broadcast sub-codeword sees independent channel with that seen by relay sub-codeword. In the broadcast phase, there’s no change to the transmission model comparing with (2.5), so it can be written as ⎧⎪r1( b ) = h1(b ) Es c1( b ) + n1(b ) ⎨ (b) (b ) (b) (b ) ⎪⎩r2 = h2 Es c 2 + n 2. (3.1). In the relay phase, Alamouti space-time code is applied, the code matrix of the ith element of the sub-codeword is. 17.
(29) ( ) ( ). ⎡c( r ) ⎢ 1,i Ci = ⎢ (r) ⎢⎣ c2,i. ∗ − c2,( ri) ⎤ ⎥ ∗ ⎥ r c1,( i ) ⎥ ⎦. (3.2). where the subscript i denotes the ith element. ( c1,( ri ) is the ith element of the vector c1( r ) ). Thus the received signal during relay phase is. ⎧ (r ) Es ( r ) ( r ) Es ( r ) c1,i + h2( r ) c2,i + n1,( ri ) ⎪r1,i = h1 2 2 ⎪ ⎨ ⎪ r ( r ) = − h ( r ) Es c ( r ) ∗ + h ( r ) E s c ( r ) 1 2,i 2 1,i ⎪⎩ 2,i 2 2. ( ). ( ) +n ∗. (3.3) (r ). 2,i. Define channel matrix of the relay phase as ⎡ h1( r ) Hr = ⎢ r ⎢ h2( ) ⎣. h2(. ( ). r). ⎤ ⎥ ∗ ⎥ ⎦. (3.4). ( ). ∗. − h1(. r). then (3.3) can be written in matrix form (r ). ri. =. =. where ri( r ) = ⎡ r1,(ir ) ⎢⎣. ( ). ⎡ (r ) Es ⎢ h1 2 ⎢ h2( r ) ⎣. h2(. ( ). ∗. r). ⎤ ⎡c ( r ) ⎤ ⎡ n1,( ri ) ⎤ ⎥ 1,i ⎥ + ⎢ ⎥ ∗ ⎢ ∗ ⎥ ⎢c2,( ri) ⎥ ⎢ n2,( ri) ⎥ ⎦⎣ ⎦ ⎣ ⎦. ( )). − h1(. r. ( ). (3.5). Es H r ci( r ) + n(i r ) 2 T. ∗ r2,( ri ) ⎤ , c(i r ) = ⎡⎣c1,( ri ) ⎥⎦. c2,( ri) ⎤⎦ , n(i r ) = ⎡ n1,( ri ) ⎢⎣ T. ( ). T. ∗ n2,( ri) ⎤ . Multiplying ⎥⎦. (3.5) by H rH , we have (r ) (r ) r i = ⎡ r 1,i ⎢⎣. =. T. (r) r 2,i ⎤ = H rH ri( r ) ⎥⎦. ⎡ h( r ) 2 + h( r ) 2 2 Es ⎢ 1 2 ⎢ 0 ⎢⎣. ⎤ (r ) ⎥ ⎡c1,i ⎤ ( r ) ⎢ r ⎥ + ni (r) 2 ( r ) 2 ⎥ ⎢c( ) ⎥ h1 + h2 ⎥ ⎣ 2,i ⎦ ⎦ 0. (3.6). (r ) (r ) where n i = H rH n i( r ) . Note that r i is a vector which contains the ith detected. symbols of relay sub-codewords for both users. Assuming ML detection, from (3.1) and (3.6) we can write the detected signals 18.
(30) for user U u ( u ∈ {1, 2} ) in broadcast phase as. ( ). (b) z u( b ) = hu( b ) ru( b ) = Es hu(b ) cu( b ) + n u ∗. 2. (3.7). ( ). (b ) where n u = hu( b ) nu(b ) . Also, the detected signal in relay phase is ∗. z u( r ) = = where h( r ) = ⎡⎣ h1( r ). (. Es ( r ) 2 h1 + h2( r ) 2 Es ( r ) h 2. 2 F. 2. )c. (r ) u. (r ). + n u. (3.8). (r). cu( r ) + nu. h2( r ) ⎤⎦ . Base codeword can be rebuilt by combing the detected T. signals. Consider the case of more than two users. For example, a 4 × 4 orthogonal space-time block code (OSTBC) can be used in 4-user coded cooperation, the protocol. is shown below: user1 data. user3 data. user2 data. user4 data. (antenna). user1 user2 user3 user4 time. . broadcast phase relay phase Fig. 3-2. Channel use of ST-coded cooperation with four users (TDMA) The major advantage of ST-coded cooperation is that space-time code with higher diversity order can be applied when more users are involved. There is no loss of. 19.
(31) spectral efficiency as long as the space-time code used is of rate equal or higher than 1.. 3.1.2 Case of Data Exchange Failure For a wireless cooperative communication system, it is always possible that data exchanged between two users are corrupted due to deep fading and cannot be decoded successfully. We call this event data exchange failure. The effect of data exchange failure to the coded cooperation can not be ignored. When it happens, cooperation can not be done since users don’t know each other’s information. Both users have to transmit their own relay sub-codewords by themselves (no cooperation mode). More specifically, if user U 2 can’t decode the broadcast sub-codeword from U1 correctly, it will notify U1 by one bit of information to let U1 transmit its relay sub-codeword itself. Meanwhile, U 2 will also transmit its relay sub-codeword itself. Cyclic redundancy check (CRC) can be used for error detection. Consider a 4-user scheme using a 4 × 4 OSTBC. Note that every user has to know the data of all other users, that is, all users have to successfully decode the data from other users in order to perform cooperation. That gives rather high requirements for inter-user channels. The effect of data exchange failure to the overall performance will be analyzed in next Section.. 3.2 Performance Bounds of ST-Coded Cooperation We present an analytical methodology in this Section for evaluating performance 20.
(32) of the proposed Alamouti ST-coded cooperation protocol. Only the performance of user U1 data is considered since the error rates of the two user data are statistically equal. The pairwise error probability is calculated using the technique from Simon and Alouini [22], then we determine the union bounds for the overall bit error rate (BER) and frame error rate (FER) using weight enumerating function and the tools given by E. Malkamäki and H. Leib [25]. These bounds will be shown and compared with the simulation result.. 3.2.1 Pairwise Error Probability Since we focus on the performance of single user throughout this Section, some notations in the equations can be simplified. Thus we can rewrite the detected signal for U1 in broadcast phase as (from (3.7)) (b ) zi( b ) = h1( b ) Es ci( b ) + n i. (3.9). where i = 1,", N b . The index i denotes the ith element of the detected signal. From (3.6), the detected signal for U1 in relay phase can be rewritten as zi( ) = r. Es ( r ) h 2. 2 F. (r ). ci( ) + n i r. (3.10). where i = 1,", N r . Sub-codewords are rearranged according to the puncturing pattern and are combined at the destination to rebuild the base codeword. The base codeword can be represented as ⎧⎪ z (b ) , z=⎨ r ( ) ⎪⎩ z ,. when i ∈ χ b when i ∈ χ r. (3.11). Note that χ b , χ r are the sets of indexes which belong to the broadcast and the relay sub-codewords, respectively. Let. γ b , γ r be the received SNRs for broadcast and relay sub-codewords, 21.
(33) respectively. It is straightforward to find that 2 ⎧ h1(b ) Es ⎪γ = ⎪⎪ b N0 ⎨ 2 ⎪ h( r ) Es F ⎪γ r = 2 N ⎪⎩ 0. (3.12). Since ML detection is used at the receiver, the corresponding symbol error probability is given by [23] 2 ⎧ ⎛ i eQ ⎜ γ b d min ⎪N ⎜ 2 ⎪⎪ ⎝ Ps ( i ) ≈ ⎨ 2 ⎪ i ⎛ γ r d min ⎪ N eQ ⎜⎜ 2 ⎪⎩ ⎝. ⎞ ⎟, ⎟ ⎠. when i ∈ χ b. ⎞ ⎟, ⎟ ⎠. when i ∈ χ r. (3.13). i e is the number of nearest neighbors and d where N min is the minimum distance of. separation of the underlying scalar constellation. Since BPSK modulation is assumed, i e = 1 and d 2 = 4 we have N min. Define the transmitted base codeword as x = [ x1 , x2 ," , xN ] , the probability that. zi is decided as an erroneous symbol xli ≠ xi conditioned on known channel ⎡ h1(b ) H=⎢ b ⎢⎣ h2( ). h1( r ) ⎤ (b) ⎥ = ⎡⎣h (r) h2 ⎥⎦. h( r ) ⎤⎦ is. ⎧ ⎛ 2 Es ( b ) 2 ⎞ h1 ⎟ , ⎪Q ⎜⎜ ⎟ N0 ⎪ ⎝ ⎠ l l P zi → xi , xi ≠ xi H, xi = Pe ≈ ⎨ ⎪ ⎛ Es ( r ) 2 ⎞ ⎪Q ⎜⎜ N h F ⎟⎟ , 0 ⎠ ⎩ ⎝. (. ). when i ∈ χ b (3.14) when i ∈ χ r. Base on above equation, we have the conditioned pairwise error probability:. ⎛ E ⎛ (b) 2 (r ) s P x ≠ x H, x = Q ⎜ ⎜⎜ ∑ 2 h1 + ∑ h ⎜ N 0 ⎝ i∈ηb i∈η r ⎝. (. where. ). ⎞⎞ ⎟⎟ F ⎟⎟ ⎠⎠. 2. (3.15). ηb is the sub set of i ∈ χb that xli ≠ xi and ηr is the sub set of i ∈ χ r 22.
(34) l ≠ x . Thus the size of that x i i. ηb are equal to the Hamming distance between the. and x . In the same way, the size of broadcast sub-codeword in x. ηr are equal to. and x . the Hamming distance between the relay sub-codeword in x Eq. (3.15) can be further simplified to 2 ⎛ E ⎛ ⎞ (b ) 2 (r) 2 ⎞ s P x ≠ x H, x = Q ⎜ d h d h + 2 ∑ r u ⎟ ⎟⎟ ⎜ N 0 ⎜⎝ b 1 u =1 ⎠⎠ ⎝. (. ). where db , d r are the sizes of. (3.16). ηb and ηr , respectively.. Since Q function can be replaced by exponential form: Q ( x) =. 1. π∫. π. 2 0. ⎛ x2 exp ⎜ − 2 ⎝ 2sin θ. ⎞ ⎟ dθ ⎠. (3.17). we have 2 ⎛ Es ⎛ (b) 2 (r ) 2 ⎞ ⎞ + d h d 2 ⎜ b 1 r ∑ hu ⎜ ⎟⎟ π N0 ⎝ 1 2 u =1 ⎠ ⎟ dθ ⎜ P x ≠ x H, x = ∫ exp − 2 0 ⎜ ⎟ 2sin θ π ⎜ ⎟ ⎝ ⎠. (. ). ⎛ E d h( b ) 2 ⎞ 2 ⎛ E d h( r ) 2 s b 1 1 ⎟ exp ⎜ − s r u = ∫ 2 exp ⎜⎜ − 2 ⎟∏ ⎜ 2 N sin 2 θ 0 π 0 ⎜ N 0 sin θ ⎟ u =1 ⎜ ⎝ ⎠ ⎝ π. (3.18) ⎞ ⎟dθ ⎟ ⎟ ⎠. Hence, the pairwise error probability is ⎛ E d h( b ) 2 ⎞ 2 ⎛ E d h( r ) 2 ⎞ π s b 1 s r u 1 ⎜ ⎟ ⎟dθ P x ≠ x x = ∫ 2 exp ⎜ − exp ⎜⎜ − ∏ 2 2 ⎟ ⎟ π 0 ⎜ N 0 sin θ ⎟ u =1 ⎜ 2 N 0 sin θ ⎟ ⎝ ⎠ ⎝ ⎠. (. ). H. (3.19). The overbar denotes statistical averaging over the random variable. Since channels between the destination and the users are assumed independent, the averaging can be performed separately, thus. 23.
(35) ⎛ E d h( b ) 2 ⎞ π s b 1 1 ⎟ P x ≠ x x = ∫ 2 exp ⎜⎜ − 2 ⎟ 0 π ⎜ N 0 sin θ ⎟ ⎝ ⎠. (. b h1( ). ). ⎛ E d h( r ) 2 ⎞ 2 s r u ⎟ exp ⎜⎜ − ∏ 2 ⎟ u =1 ⎜ 2 N 0 sin θ ⎟ ⎝ ⎠. r hu( ). dθ. (3.20). Define a random variable α that is statistically equal to the channel coefficient h1(b ) , the first term in (3.20) which averages h1(b ) over a exponential function can be written as ⎛ E d h( b ) 2 ⎞ s b 1 ⎟ exp ⎜⎜ − 2 ⎟ ⎜ N 0 sin θ ⎟ ⎝ ⎠. b h1( ). α. ⎛ E d α2 ⎞ = exp ⎜ − s b 2 ⎟ ⎝ N 0 sin θ ⎠. ∞ ⎛ E d α2 = ∫ exp ⎜ − s b 2 0 ⎝ N 0 sin θ. (3.21) ⎞ ⎟ Pα (α ) dα ⎠. Now let γ be the instantaneous received SNR (i.e. γ = α 2 Es / N 0 ), we have α. ∞ ⎛ E d α2 ⎞ ⎛ dγ exp ⎜ − s b 2 ⎟ = ∫ exp ⎜ − b2 0 ⎝ sin θ ⎝ N 0 sin θ ⎠. ⎞ ⎟ Pγ ( γ ) d γ ⎠. (3.22). The integral above is in the form of a Laplace transform of Pγ ( γ ) and is the moment generating function (MGF) of γ , thus (3.22) can be written as α. ⎛ E d α2 ⎞ exp ⎜ − s b 2 ⎟ = M γ ⎝ N 0 sin θ ⎠. db ⎞ ⎛ ⎜ − sin 2 θ ⎟ ⎝ ⎠. (3.23). In Rayleigh fading channel, the PDF of instantaneous SNR per bit is Pγ ( γ ) =. ⎛ γ exp ⎜ − γ ⎝ γ 1. ⎞ ⎟, ⎠. γ ≥0. (3.24). where γ is the average SNR per bit. The Laplace transform of the PDF is M γ ( −s ) =. 1 , 1 + sγ. Substituting (3.25) into (3.23), we have. 24. s>0. (3.25).
(36) α. ⎛ ⎛ E d α2 ⎞ d γ ⎞ exp ⎜ − s b 2 ⎟ = ⎜1 + b2 ⎟ ⎝ N 0 sin θ ⎠ ⎝ sin θ ⎠. −1. (3.26). In the same way, the other term of (3.20) can be evaluated: ⎛ E d h( r ) 2 ⎞ s r u ⎟ exp ⎜⎜ − 2 ⎟ ⎜ 2 N 0 sin θ ⎟ ⎝ ⎠. r hu( ). α. ⎛ ⎛ Es d r α 2 ⎞ dr γ ⎞ = exp ⎜ − ⎟ ⎟ = ⎜1 + 2 2 ⎝ 2 N 0 sin θ ⎠ ⎝ 2sin θ ⎠. −1. (3.27). Substituting (3.26), (3.27) into (3.20) and note that the average SNR in different (b ). (r). (r). channels may not be the same, thus we separate it by γ 1 , γ 1 , γ 2 , which represent the average SNR between user U1 and destination in broadcast phase, and the average SNR between U1 , U 2 and destination in relay phase, respectively. Thus we have (b) 1 2 ⎛ db γ 1 ⎞ ⎟ P x ≠ x x = ∫ ⎜1 + π 0 ⎜ sin 2 θ ⎟ ⎝ ⎠. (. ). π. −1. (r) ⎛ dr γ 1 ⎜1 + ⎜ 2sin 2 θ ⎝. ⎞ ⎟ ⎟ ⎠. −1. (r) ⎛ dr γ 2 ⎜1 + ⎜ 2sin 2 θ ⎝. −1. ⎞ ⎟ dθ ⎟ ⎠. (3.28). From (3.28) it is clear that a diversity order of 3 is achieved as long as db , d r ≠ 0 , that is, the Hamming distances between broadcast, relay sub-codewords and the transmitted sub-codewords are not zero. The diversity is gained by the use of Alamouti space-time code in the relay sub-codeword, and by the independent channel seen in broadcast phase.. 3.2.2 Bit and Block Error Rate A union bound for the BER and FER can be calculated using weight enumerating functions. In traditional approach [24], the first step is finding the first-error-event probability. Assume all-zero path is the correct path, we want to find the probability that a path through the trellis with Hamming distance d from the all-zero path is the survivor. The second step is taking the summation of the first-error-event probabilities 25.
(37) over all possible Hamming distances. Note the Hamming distance between a path and all-zero path is also the weight of that path. Recall that (3.28) is a function of (b ). (r ). (r ). Hamming distances db , d r and average SNRs γ 1 , γ 1 , γ 2 . Now we assume the SNRs are given, (3.28) can be rewritten as a pairwise error probability function of only the Hamming distances. (. P2 ( db , d r ) = P x ≠ x x , γ (b ). (r). where γ is a SNR vector with elements γ 1 , γ 1. ). (3.29) (r). and γ 2 . Denoting the number of. paths that the broadcast and relay sub-codewords have weights db , d r by a ( db , d r ) , we can bound the first-error-event probability by ∞. ∞. Pe ≤ ∑ ∑ a ( db , d r ) P2 ( db , d r ). (3.30). d1 =1 d 2 =1. where on the right-hand side, we have included all paths through the trellis that merge with the all-zero path. To calculate BER, first define b ( db , d r ) as the total number of bit errors in paths that the broadcast and relay sub-codewords have weights db , d r , then the union bound of BER is ∞. ∞. Pb ≤ ∑ ∑ b ( db , d r ) P2 ( db , d r ). (3.31). db =1 d r =1. However, it is shown in [25] that the union bound approach was found to provide quite loose bounds. This is because there is no dominant error event in (3.31), even at high SNR region. Therefore, a modification to this method is proposed by [25] to obtain a much tighter bound. It is done by limiting the conditioned union bound on the bit error probability before averaging over the fading matrix. Thus we need the pairwise error probability conditioned on a given channel, which is given in (3.18). Rewrite (3.18) as 26.
(38) P2 (α1 , α 2 , α 3 , db , d r ) =. 1. π. ∫. π. 2 0. ⎛ Es dbα12 ⎞ ⎛ ⎛ Es d rα 22 ⎞ Es d rα 32 ⎞ exp ⎜ − ⎟ exp ⎜ − ⎟ exp ⎜ − ⎟ dθ 2 2 2 ⎝ N 0 sin θ ⎠ ⎝ 2 N 0 sin θ ⎠ ⎝ 2 N 0 sin θ ⎠. (3.32) where α1 = h1( b ) , α 2 = h1( r ) , α 3 = h2( r ) . Then we sum over all possible distances to obtain the bound of bit error probability which is a function of known channel coefficients:. ⎡1 ∞ ∞ ⎤ Pb (α1 , α 2 , α 3 ) ≤ min ⎢ , ∑ ∑ b ( db , d r ) P2 (α1 , α 2 , α 3 , db , d r ) ⎥ ⎣ 2 db =1 dr =1 ⎦. (3.33). The bit error probability is limited to 1/2 because in practice, the maximum error rate using Viterbi decoder is 1/2. By averaging over the channel coefficients, the new bound can be obtained:. Pb ≤ ∫. ∫ ∫. α1 α 2 α 3. Pb (α1 , α 2 , α 3 ) Pα (α1 ) Pα (α 2 ) Pα (α 3 ) dα 3 dα 2 dα1. (3.34). A much tighter bound can be obtained this way, but the order of the integrations and the summations can not be exchanged in (3.34) due to the min operator, thus numerical integral is needed to calculate the results. The bounds will be compared with simulation results in Section 3.3. Frame error rate can be evaluated in similar way. For a data block of K bits, given the event error probability Pe , the FER can be bounded as [26]. Pf ≤ 1 − (1 − Pe ) ≤ KPe K. The. traditional. approach. gives. loose. bound. (3.35) for. the. FER,. thus. the. limit-before-averaging technique is applied. We first calculate the bound of event error probability conditioned on given channel coefficients:. ⎡ ∞ ∞ ⎤ Pe (α1 , α 2 , α 3 ) ≤ min ⎢1, ∑ ∑ a ( db , d r ) P2 (α1 , α 2 , α 3 , db , d r ) ⎥ ⎣ db =1 dr =1 ⎦ 27. (3.36).
(39) This time the event error rate is limited to 1 because the maximum error rate is 1 in practice. Apply (3.36) to (3.35) and average over the channel coefficients we get. (1 − P (α , α ,α ) ) α ∫α ∫α. Pf ≤ 1 − ∫. e. 1. 2. 1. 2. K. 3. Pα (α1 ) Pα (α 2 ) Pα (α 3 ) dα 3 dα 2 dα1 (3.37). 3. Again, the above equation needs to be carried out numerically in this case due to the min operator.. 3.2.3 Impact of Data Exchange Failure Now consider the impact to the performance due to data exchange failure. We focus on 2-user case first. Since the transmissions from user U1 to U 2 and from. U 2 to U1 are on the same frequency band and the same coherence interval, we assume they see equal channels due to the reciprocity theorem [27]. Denoting Pf ,u as the rate of the data exchange failure between U1 and U 2 , the probability of successfully cooperation between two users can be written as. PC = 1 − Pf ,u. (3.38). Denote the FER of 2-user cooperation with no data exchange failure as Pf ,2user and the FER which data exchange always fails as Pf ,nocoop . We have shown how to calculate Pf ,2user in previous two Sections. The calculation of Pf ,nocoop can be done simply by setting h2( r ) to h1( r ) in (3.18). Thus the overall frame error rate for U1 data is. Pf = Pf ,2user PC + Pf , nocoop (1 − PC ) = Pf ,2user (1 − Pf ,u ) + Pf ,nocoop Pf ,u. (3.39). Since Pf ,2user has diversity order of 3 but Pf ,nocoop has only 2, at high SNR region,. 28.
(40) the frame error rate will be dominated by Pf ,nocoop and (3.39) can be bounded as. Pf > Pf ,nocoop Pf ,u. (3.40). It can be seen that the diversity gain decreases at high SNR region. Now consider a 4-user scheme. Note that all users have to decode data from others correctly to apply 4 × 4 OSTBC. Assuming the rates of data exchange failure are equal between all users, the probability of successfully cooperation can be written as. PC ,4 = (1 − Pf ,u ). 6. (3.41). Thus we have the overall FER in 4-user case as Pf = Pf ,4user PC ,4 + Pf ,nocoop (1 − PC ,4 ). (. = Pf ,4user (1 − Pf ,u ) + Pf ,nocoop 1 − (1 − Pf ,u ) 6. 6. ). (3.42). where Pf ,4user is the FER with 4-user coded cooperation and no data exchange failure. The overall FER can be bounded as follow in high SNR region. (. Pf > Pf , nocoop 1 − (1 − Pf ,u ). 6. ). (3.43). Again, we can expect the loss in diversity order at high SNR region, what’s more,. (. note that 1 − (1 − Pf ,u ). 6. ). is much higher than Pf ,u , the degradation of performance. will be larger compared to 2-user case. It will be shown in Fig. 3-4 in next Section. To this problem, an adaptive scheme can be used to compensate the huge performance loss in 4-user case. It is unnecessary to make all users back to no cooperation mode if some of them still received others’ data correctly. Instead, other space-time codes can be applied to the users who is capable for cooperation. For example, if there are only two users successfully exchanged information with each other, an Alamouti space-time code is applied instead of 4 × 4 OSTBC; if there are three, a 3-user space-time code can be used. For simplicity, consider only using 29.
(41) Alamouti code and 4 × 4 OSTBC in the adaptive scheme, the probability of frame error becomes. Pf = Pf ,4user PC ,4 + Pf ,2user PC ,2 + Pf ,nocoop PC ,0. (3.44). where PC ,4 is the probability that all four users exchanged information successfully, which is equal to (3.41); PC ,2 is the probability of any user successfully exchanged data with U1 ; PC ,0 is the probability of no user successfully exchanged data with. U1 . They are PC ,0 = Pf3,u PC ,4 = (1 − Pf ,u ). 6. (3.45). PC ,2 = 1 − PC ,4 − PC ,0. respectively. Thus (3.44) can be written as. (. ). Pf = Pf ,4user (1 − Pf ,u ) + Pf ,2user 1 − (1 − Pf ,u ) − Pf3,u + Pf , nocoop Pf3,u 6. 6. (3.46). The FER at high SNR region can be bounded as Pf ≈ Pf ,nocoop Pf3,u. (3.47). Note the difference between (3.43) and (3.47). The probability that users go back to no cooperation mode is much lower comparing to the probability in non-adaptive scheme, thus provides a better performance. Simulations of the adaptive scheme will be presented in Fig. 3-5 in next Section.. 3.3 Computer Simulations In this Section we simulate the proposed space-time coded cooperation protocols and compare with other protocols mentioned in Chapter 2. All systems are with equal code rate R and hence equal data rate. We use a rate-1/4 base code with generator [15 30.
(42) 17 13 15] used by [18]. For the conventional coded cooperation and the proposed ST-coded cooperation, the puncture patterns are [1 1 0 0] for the broadcast sub-codeword, [0 0 1 1] for the relay sub-codeword. Binary phase-shift keying (BPSK) modulation is used. The frame size is 260 bits. We consider the case that both nodes communicate with the same destination. Each user and the destination are equipped with a single antenna. The channel is slow Rayleigh fading channel with AWGN. Fig. 3-3 plots the analytical bound and simulation results of FER as a function of the transmit SNR. Similar results could be obtained in terms of BER. Perfect inter-user channel is assumed so there is no data exchange failure. Investigating the FER at high SNR region and comparing with the analytical bounds (dash line with diamonds), it is clear that the proposed ST-coded cooperation (line with diamonds) achieves full diversity as we expected in Section 3.2. Comparing the performance of the proposed protocol with conventional coded cooperation (line with squares) at FER of 10−3 , the proposed ST-coded cooperation using Alamouti code achieves nearly 4dB gain over the conventional coded cooperation. If more users join the cooperation, higher order space-time code can be used to gain more diversity. For the case of four users (line with down triangles), additional gain of 2.5dB is achieved comparing to 2-user case.. 31.
(43) 10. 10. FER. 10. 10. 10. 10. 10. 0. Direct transmission Conventional coded cooperation (union bound) Conventional coded cooperation Proposed ST-coded (Alamouti)(union bound) Proposed ST-coded (Alamouti) Proposed ST-coded (4x4 OSTBC)(union bound) Proposed ST-coded (4x4 OSTBC). -1. -2. -3. -4. -5. -6. 0. 2. 4. 6. 8. 10 SNR (dB). 12. 14. 16. 18. 20. Fig. 3-3. Simulations and bounds of frame error rate (FER) in ST-coded cooperation. Equal uplink SNR, base code [15 17 13 15] Fig. 3-4 shows the impact of data exchange failure to the overall FER. We assume that the rate of data exchange failure between users is 0.1. Analytical bounds based on Section 3.2.3 are shown in the figure. It can be seen that the simulation result is consistent with the analytical bound. Lines with diamonds and down triangles are the same as the simulation results in Fig. 3-3, that is, ST-coded cooperation with no data exchange failure. Diamonds and down triangles with no lines are the simulation result when data exchange failure is considered. From the figure we can see that the proposed ST-coded cooperation protocols lose their diversity at high SNR region. At that region, they seem to have diversity of order 2 because the two sub-codewords still experience independent. 32.
(44) channels in broadcast and relay phase. Despite the loss in diversity, the proposed 2-user ST-coded cooperation still has advantages over the conventional one. But the performance of 4-user scheme is even worse than 2-user case. As mentioned in Section 3.2.3, cooperation among four users with imperfect inter-user channel experiences severe performance degradation since the data exchange between 4 users is hardly all successful. To this problem, we’ll show in Fig. 3-5 the performance improvements of 4-user cooperation with adaptive protocol.. 10. 10. FER. 10. 10. 10. 10. 10. Direct transmission Conventional coded cooperation (failure rate=0.1) ST-coded (4x4 OSTBC) (failure rate=0.1) (union bound) ST-coded (4x4 OSTBC) (failure rate=0.1) ST-coded (Alamouti) (failure rate=0.1) (union bound) ST-coded (Alamouti) (failure rate=0.1) ST-coded (Alamouti) (perfect cooperation) ST-coded (4x4 OSTBC) (perfect cooperation). 0. -1. -2. -3. -4. -5. -6. 0. 2. 4. 6. 8. 10 SNR (dB). 12. 14. 16. 18. 20. Fig. 3-4. Frame error rate (FER) with imperfect inter-user channels. Equal uplink SNR, generator [15 17 13 15], inter-user FER=0.1 Comparing the performance of 4-user case (down triangles) in Fig. 3-4 and Fig. 3-5, we can see clearly the improvement by applying adaptive protocol mentioned in. 33.
(45) Section 3.2.3. The algorithm assures the performance under 4-user scheme not worse than the case when only 2-user Alamouti code is applied. Meanwhile, it still benefits from the use of 4 × 4 OSTBC when the data is exchanged successfully. For the case of data exchange failure rate=0.1, the average probability of 4-user cooperation is about 0.531, 2-user cooperation is about 0.468 and the probability of no cooperation is only 0.001.. 10. 10. FER. 10. 10. 10. 10. 10. 0. Direct transmission Conventional coded cooperation (inter-user FER=0.1) ST-coded (Alamouti) (failure rate=0.1) ST-coded (Alamouti) (perfect cooperation) ST-coded (4x4 OSTBC) (failure rate=0.1) (union bound) ST-coded (4x4 OSTBC) (failure rate=0.1) ST-coded (4x4 OSTBC) (perfect cooperation). -1. -2. -3. -4. -5. -6. 0. 2. 4. 6. 8. 10 SNR (dB). 12. 14. 16. 18. 20. Fig. 3-5. Frame error rate (FER) with imperfect inter-user channels. Equal uplink SNR, generator [15 17 13 15], inter-user FER=0.1, adaptive algorithm. 3.4 Summary In this Chapter we give a detailed description of the proposed space-time coded 34.
(46) cooperation protocol. We show the diversity gain in the case of 2-user coded cooperation with Alamouti space-time code by evaluating the pairwise error probability. Extension to other space-time code is straightforward. The proposed protocol can utilizes full diversity gain from the used space-time code. Tight union bounds for the BER as well as the FER are given by using the weight enumerating function and the limit-before-averaging technique. Both analytical and simulation results have been shown to prove the performance gain. We also consider the impact of imperfect inter-user channel to the proposed protocol and give an adaptive way to reduce the performance loss.. 35.
(47) Chapter 4 Spectrally Efficient Multi-user Coded Cooperation using Code Partitioning In this Chapter we introduce the second modification of coded cooperation: code-partition (CP) coded cooperation. The proposed protocols still achieve great system reliability while maintaining equal spectral efficiency as non-cooperation protocols. The CP-coded cooperation has similar performance to the protocols in Chapter 3. Besides, it has the advantages of less complexity and lower requirements for inter-user channel.. 4.1 Protocols of CP-Coded Cooperation Look at the performance bound in eq. (3.28), we can see that the diversity gain comes from the independent channels of user U1 in the broadcast phase and relay phase. Further more, additional diversity is gained from U 2 channel by using Alamouti space-time code in relay phase. Thus the total diversity gain compared to direct transmission protocol is 2 + 1 = 3 . There is another factor in (3.28) that contributes to the overall performance: the Hamming weight of the sub-codewords 36.
(48) ( d b , d r ). As long as d b , d r are not zeros, diversity order of 3 is achieved. Since that, it is natural to think that if more than two sub-codes are separated from the base code and transmitted by independent channels, higher diversity order can be achieved without the help of space-time code. The second type of coded cooperation is proposed based on these principles.. 4.1.1 Code Structure and System Model The main idea of CP-coded cooperation is to let every sub-codeword of a base codeword transmitted through independent channels. Thus the number of sub-codes depends on the number of independent channels we have. For 2-user case, we partition the base code into three sub-codes, one is for the source and the other two are for the relays (See Fig. 4-1). The sub-codeword for the source is still called broadcast sub-codeword, but for the other two sub-codewords for the relays, we tag them with numbers (ex. 1st, 2nd relay sub-codeword, etc.), each relay sub-codewords are transmitted by different relays. The code structure is shown in Fig. 4-2.. 37.
(49) user1. user2. i. i. broadcast sub-codeword. (antenna). ith relay sub-codeword. user1. 1. user2. 1 2. 2. time. . broadcast phase relay phase Fig. 4-1. Channel use of CP-coded cooperation with two users (TDMA). base code. puncturing. 12. 12 12 12 12 12 12 12. sub-codes. 1. 12. 2. . 1st relay 2nd relay broadcast sub-codeword sub-codeword sub-codeword. Fig. 4-2. Structure of codeword in CP-coded cooperation (2-user case) Note that the code-structure is different from the one in Chapter 3 and [18]. We expect a diversity order of 3 is gained for each user data since the data is partitioned into three parts. The analysis will be given in Section 4.2. Assume quasi-static fading channel similar to the channel used in ST-coded cooperation, the received signal at the destination in broadcast phase is. 38.
(50) ⎧⎪r1( b ) = h1(b ) Es c1( b ) + n1(b ) ⎨ (b) (b) (b ) (b ) ⎪⎩r2 = h2 Es c 2 + n 2. (4.1). where c1(b ) , c(2b ) denotes the broadcast sub-codewords of user U1 and U 2 , respectively. At relay phase, the received signal can be written as ⎧⎪r1( ri ) = hi( r ) Es c1( ri ) + n1( ri ) ⎨ ( ri ) ( ri ) ( ri ) (r ) ⎪⎩r2 = hi Es c 2 + n 2. (4.2). where i = {1, 2} , c1( ri ) , c(2ri ) denotes the ith relay sub-codeword of user U1 and U 2 , respectively. Assuming ML detection, the detected signal for user U1 is ⎧ z (b ) = E h (b ) 2 c (b ) + n 1( b ) s 1 1 ⎪ 1 2 ⎪ ( r1) ( r1) (r) ( r1) ⎨ z1 = Es h1 c1 + n 1 ⎪ 2 ⎪ z1( r 2 ) = Es h2( r ) c1( r 2 ) + n 1( r 2 ) ⎩. ( ). ( ). (4.3). ( ). ∗ ∗ ∗ (b) ( r1) ( r 2) where n 1 = h1(b ) n1(b ) , n 1 = h1( r ) n1( r1) and n 1 = h1( r ) n1( r 2 ) . These detected. signals are combined at the destination to rebuild the base codeword. Then the destination decodes the base codeword using Viterbi decoder. This protocol can be easily extended to cases with more users, for example, a 4-user protocol is shown below.. 39.
(51) sub-codeword for source ith sub-codeword for relay. user1. user2. user3. i. i. i. user4. i. user1 (antenna). 1. 1. 1. 1. user2 2. 2. 2. 2. user3 3. 3. 3. 3. user4 4. 4. 4. 4. time . broadcast phase relay phase Fig. 4-3. Channel use of CP-coded cooperation with four users (TDMA). base code. puncturing. sub-codes. 1234. 1234 1234 1234 1234 1234. 1. 2. 3. 4. . 1st relay broadcast 2nd relay 3rd relay 4th relay sub-codeword sub-codeword sub-codeword sub-codeword sub-codeword. Fig. 4-4. Structure of codeword in CP-coded cooperation (4-user case) This time the base code is partitioned into five sub-codes, the first one is for broadcasting and the other four is for relaying (four relays). Note that the four relay sub-codewords are still transmitted by different users. The main difference between ST-coded cooperation and CP-coded cooperation is 40.
(52) the number of sub-codes used. In ST-coded cooperation, the number is fixed to two sub-codes: one for broadcasting and one for relaying. It is the space-time code to change with different number of users. However, CP-coded cooperation uses different number of sub-codes to match the number of users participated in the cooperation.. 4.1.2 Case of Information Exchange Failure CP-coded cooperation is more flexible than ST-coded cooperation to the case of data exchange failure; the latter needs all users to exchange data successfully to apply space-time code, while the former doesn’t. For example, in a 4-user scheme, if error occurs in the exchange of data between user U1 and U 2 , they can’t cooperate with each other so the diversity gain will be lower due to the loss of one independent channel. However, they can still cooperate with U 3 and U 4 , so it has no effect to the error rates of U 3 and U 4 data. There are two kinds of reactions for a user when decoding error occurs: notify other users (method 1) or do nothing (method 2). More specific description will be given in the following paragraph.. Method 1 The first method is the similar to the method used in ST-coded cooperation. Say, in a 4-user scheme, if user U1 fails to decode the information from U 2 , it will send a notification signal to all other users. However, there is no need for all users to go back to no cooperation mode (like we do in 3.1.2), instead, only U 2 takes this notification signal. After receiving the notification, U1 and U 2 will go back to no cooperation mode and transmit the corresponding sub-codewords by themselves. A figure plotted the channel use under above case is given in Fig. 4-5. From the figure 41.
(53) we can see that the sub-codewords (2nd sub-codeword of U1 and 1st sub-codeword of. U 2 ) which should be sent by other users are now sent by its owners themselves ( U1 , U 2 , respectively). However, there’s no change for U 3 and U 4 data. Thus full diversity order can still be achieved for these two users, while diversity of order one will be lost for U1 and U 2 data.. broadcast sub-codeword ith relay sub-codeword. (antenna). user1. user1. user2. user3. i. i. i. 12. user4. i. 1. 1. user2 2. 12. 2. user3 3. 3. 3. 3. user4 4. 4. 4. 4. time . broadcast phase relay phase Fig. 4-5. Channel use of 4-user CP-coded cooperation with bad user1 – user2 link, method 1. Method 2 The second method makes slightly modification to the first one. Since a long base codeword is partitioned into several sub-codewords, it may still be okay for the destination to decode if some of the sub-codewords are lost. That is, if a user fails to decode the information from other user in broadcast phase, it does nothing in the corresponding relay phase. The destination will hence lose that part of the base. 42.
(54) codeword, but data can still be decoded from the remaining parts. This method will lead to some loss in not only diversity gain, but also coding gain; however, the major advantage is that users don’t need to notify others if decoding error occurs, hence the system complexity is less than the first method. Fig. 4-6 gives the example when data exchange failure occurs between U1 and U 2 . Note that compared with Fig. 4-5, some sub-codewords are discarded instead of transmitted by their owner.. broadcast sub-codeword ith relay sub-codeword. (antenna). user1. user1. user2. user3. i. i. i. 1. user4. i. 1. 1. user2 2. 2. 2. user3 3. 3. 3. 3. user4 4. 4. 4. 4. time . broadcast phase relay phase Fig. 4-6. Channel use of 4-user CP-coded cooperation with bad user1 – user2 link, method 2. 4.2 Performance Bounds of CP-Coded Cooperation Using the same technique in Section 3.2, we find the diversity gain through the evaluation of the pairwise error probability of 2-user CP-coded cooperation. Let 43.
(55) N b , N r1 , N r 2 denote the length of the broadcast, 1st relay and 2nd relay sub-codewords in Fig. 4-2. From (4.3) we can rewrite the detected signal for user U1 in symbol-wise form: ⎧ z ( b ) = E h (b ) 2 c ( b ) + n i(b ) , s i 1 ⎪ i 2 ⎪ ( r1) ( r1) (r ) ( r1) ⎨ zi = Es h1 ci + n i , ⎪ 2 r2 ⎪ zi( r 2 ) = Es h2( r ) ci( r 2 ) + n i( ) , ⎩. i = 1," , N b i = 1," , N r1. (4.4). i = 1," , N r 2. where the index i denotes the ith element of the corresponding vector in (4.3). Thus the base codeword after combining is. ⎧ z (b) , ⎪⎪ z = ⎨ z ( r1) , ⎪ ( r 2) ⎪⎩ z ,. when i ∈ χ b when i ∈ χ r1. (4.5). when i ∈ χ r 2. Note that χ b , χ r1 , χ r 2 are the sets of indexes which belong to the broadcast, 1st relay and 2nd relay sub-codewords, respectively. The received SNR in the three phases are 2 ⎧ Es h1(b ) ⎪γ = ⎪ b N0 ⎪ 2 ⎪ Es h1( r ) ⎪ ⎨γ r1 = N0 ⎪ 2 ⎪ Es h2( r ) ⎪ ⎪γ r 2 = N 0 ⎪⎩. broadcast sub-codeword. 1st relay sub-codeword. (4.6). 2 nd relay sub-codeword. Hence the error probability of each symbol in (4.5) can be expressed as ⎧ ⎛ γ d2 ⎞ i ⎪ N eQ ⎜ b min ⎟ , ⎜ 2 ⎟⎠ ⎪ ⎝ ⎪ 2 ⎞ ⎪ i ⎛ γ r1d min Ps ( i ) ≈ ⎨ N eQ ⎜ ⎟, ⎜ 2 ⎟⎠ ⎪ ⎝ ⎪ 2 ⎪ i ⎛ γ r 2 d min ⎞ N Q ⎟, ⎪ e ⎜⎜ ⎟ 2 ⎝ ⎠ ⎩ 44. when i ∈ χ b when i ∈ χ r1 when i ∈ χ r 2. (4.7).
(56) i e = 1 and d 2 = 4 . Define user U ’s Again we assume BPSK modulation, thus N min 1. codeword as x = [ x1. x2 " xN ] . The symbol error probability that zi is decided. l ≠ x conditioned on known channel is as an erroneous symbol x i i ⎧ ⎛ 2 Es ( b ) 2 ⎞ h1 ⎟ ⎪Q ⎜ ⎪ ⎝ N0 ⎠ ⎪ ⎪ ⎛ 2 Es ( r ) 2 ⎞ P zi → xli , xli ≠ xi h, xi ≈ ⎨Q ⎜ h1 ⎟ ⎠ ⎪ ⎝ N0 ⎪ 2 ⎪Q ⎛⎜ 2 Es h ( r ) ⎞⎟ 2 ⎪ ⎝ N0 ⎠ ⎩. (. when i ∈ χ b. ). where the channel vector is defined as h = ⎡⎣ h1( b ). h1( r ). when i ∈ χ r1. (4.8). when i ∈ χ r 2 T. h2( r ) ⎤⎦ .. In the end, we can write the pairwise error probability of the received codeword conditioned on known channel in the form similar to (3.15) ⎛ 2E ⎛ ⎞ (b) 2 (r) 2 (r) 2 ⎞ s P x ≠ x h, x = Q ⎜ ⎜ ∑ h1 + ∑ h1 + ∑ h2 ⎟ ⎟ ⎜ N 0 ⎝ i∈ηb i∈ηr 1 i∈ηr 2 ⎠ ⎟⎠ ⎝. (. where. ). (4.9). ηb ,ηr1 and ηr 2 are the sub sets of i ∈ χ b , i ∈ χ r1 and i ∈ χ r 2 , respectively,. l ≠ x . Thus we can remove the summations in (4.9) to simplify it as we have that x i i done to (3.15): ⎛ 2 Es ⎛ (b) 2 (r) 2 (r) 2 ⎞ ⎞ P x ≠ x h, x = Q ⎜ ⎜ d b h1 + d r1 h1 + d r 2 h2 ⎟ ⎟ ⎠⎠ ⎝ N0 ⎝. (. ). where d b , d r1 , d r 2 are the sizes of. (4.10). ηb ,ηr1 and ηr 2 , respectively.. Eq. (4.10) is in similar form to (3.16), so we can apply the same simplifying technique used in Section 3.2.1 to evaluate the pairwise error probability. Thus we have. 45.
(57) ⎛ d γ (b) ⎞ 2⎜ P x ≠ x x = ∫ 1 + b 21 ⎟ π 0 ⎜ sin θ ⎟ ⎝ ⎠. (. (b). ). (r). where γ 1 , γ 1. π. 1. (r). and γ 2. −1. ⎛ d γ (r) ⎞ ⎜ 1 + r1 2 1 ⎟ ⎜ sin θ ⎟ ⎝ ⎠. −1. −1. ⎛ d γ (r) ⎞ ⎜ 1 + r 2 2 2 ⎟ dθ ⎜ sin θ ⎟ ⎝ ⎠. (4.11). represent the average SNR between user U1 and. destination at broadcast phase and between user U1 ,U 2 and destination at relay phase, respectively. It can be seen that when all of the distance parameters db , d r1 and d r 2 are not zeros, diversity order of 3 can be achieved. Diversity of more user case can be easily proved by the same method in this Section. For example, a 4-user scheme with base code partitioned into five parts has diversity order of 5 since it utilized the independent channels h1( b ) , h1( r ) , h2( r ) , h3( r ) and h4( r ) (for U1 ).. Union bounds for BER and FER of these protocols can be calculated by the same method in Section 3.2.2, so we skip this part and look directly to the case of data exchange failure.. 4.2.1 Impact of Data Exchange Failure We analyze the impact of imperfect inter-user channel to the overall performance of user U1 data. Both method 1 and method 2 in Section 4.1.2 will be considered.. Method 1 Denote Pf ,u as the rate of data exchange failure between users. In 2-user case, the probability of successful cooperation is (1 − Pf ,u ) and the probability of going back to no cooperation mode is Pf ,u . Thus the overall performance can be written as Pf = Pf ,2 user (1 − Pf ,u ) + Pf ,nocoop Pf ,u 46. (4.12).
(58) where Pf ,2user is the FER of 2-user CP-coded cooperation, which can be calculated from (4.11); Pf ,nocoop is the FER when no cooperation is performed, it can be calculated by replacing h2( r ) in (4.10) with h1( r ) , since the sub-codeword is now sent by U1 itself. Evaluating the pairwise error probability under above situation, we have. P2,nocoop. (. ⎛ d γ (b) ⎞ x ≠ x x = 2⎜ 1+ b 1 ⎟ π ∫0 ⎜ sin 2 θ ⎟ ⎝ ⎠. ). 1. π. −1. −1. ⎛ ( d + d ) γ (r) ⎞ ⎜ 1 + r1 2r 2 1 ⎟ dθ ⎜ ⎟ sin θ ⎝ ⎠. (4.13). Note that the diversity is lost comparing with (4.11). Also note that (4.12) is in similar form to (3.39), thus loss of diversity to the overall FER is expected at high SNR. In 4-user case, full diversity can be achieved for user U1 data if and only if the links between it and other three users are available. Thus the probability of 4-user cooperation is. (1 − P ) f ,u. 3. . If one of the users doesn’t decode successfully, the. situation becomes 3-user cooperation scheme and the probability of this condition is 3Pf ,u (1 − Pf ,u ) . Also, the probability of 2-user cooperation and no cooperation is 2. 3Pf2,u (1 − Pf ,u ) and Pf3,u , respectively. We denote the FER under 3-user, 2-user and no cooperation as Pf ,3user , Pf ,2user and Pf ,nocoop , respectively. It can be calculated in the same way as Pf ,nocoop in 2-user case described above. The overall FER at the destination is Pf = Pf ,4user (1 − Pf ,u ) + 3Pf ,3user Pf ,u (1 − Pf ,u ) 3. +3Pf ,2user Pf2,u (1 − Pf ,u ) + Pf ,nocoop Pf3,u. 2. (4.14). Method 2 The only difference between method 1 and method 2 is the value of 47.
(59) Pf ,3user , Pf ,2user and Pf ,nocoop ; since some of the codewords are discarded in method 2, it will lose some coding gain. The overall FER is calculated using the same equations as method 1, that is, (4.12) for 2-user case and (4.14) for 4-user case. Consider 2-user case for instance, Pf ,nocoop is calculated simply by setting the ⎛ d γ (r) term ⎜1 + r 2 2 2 ⎜ sin θ ⎝. ⎞ ⎟ ⎟ ⎠. −1. in (4.11) to 1, because the 2nd sub-codeword is discarded. Thus. we have P2,nocoop. (. ⎛ d γ (b) ⎞ 2⎜ x → x = ∫ 1 + b 21 ⎟ π 0 ⎜ sin θ ⎟ ⎝ ⎠. ). 1. π. −1. −1. ⎛ d γ (r) ⎞ ⎜1 + r1 2 1 ⎟ dθ ⎜ sin θ ⎟ ⎝ ⎠. (4.15). Note the difference between (4.13) and (4.15). The distance value in the second term is changed from. ( d r1 + d r 2 ). to d r1 , this is because the sub-codeword for U 2 is. discarded instead of transmitted by U1 . The difference between (4.13) and (4.15) implies loss of coding gain when method 2 is used, but the diversity gain is preserved.. 4.3 Computer Simulations We now simulate the proposed CP-coded cooperation protocols and compare them with the performance bounds. Two base codes will be used: [15 17 13 15] (rate-1/4) and [15 17 13 15 13 17] (rate-1/6). The constraint length is 4 and the frame size is 260 bits. 2-user and 4-user cases will be considered; all users are equipped with single antenna and are communicating with the same destination. To isolate the diversity gain from the cooperation, the destination is equipped with only one antenna. However, more antennas can be used to further enhance the system reliability.. 48.
數據

![Fig. 1-2. System design of (a) Network coding [13], (b) Network coding [14], (c) Spatial reuse [15], (d) Coded cooperation[16]](https://thumb-ap.123doks.com/thumbv2/9libinfo/8466181.183358/14.892.184.729.530.1029/design-network-coding-network-coding-spatial-coded-cooperation.webp)
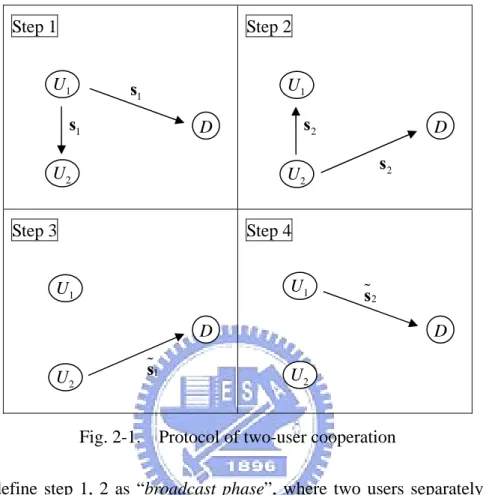
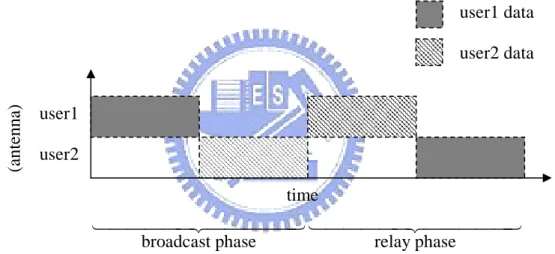
相關文件
Exploit antenna diversity to increase the SNR of a single stream. Receive diversity and
Exploit antenna diversity to increase the SNR of a single stream. Receive diversity and
When we know that a relation R is a partial order on a set A, we can eliminate the loops at the vertices of its digraph .Since R is also transitive , having the edges (1, 2) and (2,
• Content demands – Awareness that in different countries the weather is different and we need to wear different clothes / also culture. impacts on the clothing
Guiding students to analyse the language features and the rhetorical structure of the text in relation to its purpose along the genre egg model for content
We explicitly saw the dimensional reason for the occurrence of the magnetic catalysis on the basis of the scaling argument. However, the precise form of gap depends
Miroslav Fiedler, Praha, Algebraic connectivity of graphs, Czechoslovak Mathematical Journal 23 (98) 1973,
Moreover, for the merit functions induced by them for the second-order cone complementarity problem (SOCCP), we provide a condition for each stationary point being a solution of