超寬頻次諧波正交中頻訊號混頻器與鏡像消除混頻器
85
0
0
全文
(2)
(3) 䟚£>2ämU¹äÂDŸd¾Î¹ä UWB Subharmonic Quadrature-IF Mixer and Image Rejection Mixer. û. ˝. Þ : ’Ì. Student : Chih-Hao Yang. N û ` ¤ : "/± ²=. Advisor : Dr. Chi-Yang Chang. Å > ¦ × ç Ú ] ˙ ç Í î=d. A Thesis Submitted to Institude of Communication Engineering College of Electrical Engineering and Computer Science National Chiao Tung University in Partial Fulfillment of the Requirements for the Degree of Master of Science in Communication Engineering June 2008 HsinChu, Taiwan, Republic of China. 2M¬Å þý~.
(4)
(5) 䟚£>2ämU¹äÂDŸd¾Î¹ä N û ` ¤: "/± ²=. û˝Þ: ’Ì. Å >¦×çÚ] ˙û˝F b. ¿. Ì(¦m2í äÍ$, MÚÆ‰AÑø_ÏÆ1/½bí¦mÍ$, äÍ$íÔ4Ê kË'×íä £0§íf§0 ¾Î äÍ$¹äÂ52íŸdmU, uTôÍ$4 ?í^j¶ 7Ê äÍ$¹äÂç2, ä 90¯Âu|½bíjK …d퇚¨, Bb}UàÖ-ZVõÛø_ äí90¯Â, w2¯# |#í¶M, }‚àò¯ÂV®A ?Óª¯Â¥¦¸D½"4^?í^k-Z6 }ʤ¹d2n …dí(š¨}Üs¹ä øus_|Î5È} 90 óPÏퟚ £>2ämU¹äÂ, ù†ux¾ÎŸdmUŠ?ퟚ¹ä œüíÞ Uà œýíjKb, u¥s¹äÂíiõ. i.
(6) ii.
(7) UWB Subharmonic Quadrature-IF Mixer and Image Rejection Mixer Student: Chih-Hao Yang. Advisor: Dr. Chi-Yang Chang. Institude of Communication Engineering National Chiao Tung University Abstract The UWB system is receiving growing attention as an important communication system for wireless communication. The characteristics of UWB system are the ultra wide bandwidth and its high data rate transmission. In order to obtain a better performance of the UWB system, an UWB mixer with the function of rejecting image signal is needed. An ultra broadband directional coupler is the most important element of the UWB mixer due to the large bandwidth. In first part of this thesis, we will utilize multisection structure to perform an UWB coupler. The most tightly coupling section of the multisection coupler is fabricated by VIP structure. The compensated stub, which can improve return loss and isolation of this coupler, is presented in this thesis. In the second part of this thesis, two kinds of mixers are discussed. The first one is a subharmonic quadrature-IF mixer, the phase difference between two output ports is 90 degree. The second one is a subharmonic image rejection mixer. All the mixers mentioned above have the benefits of small layout area and fewer elements.. iii.
(8) iv.
(9) Acknowledgement. Ðá. ÊBíÞ·2rÖA, Fbí|Û, UBí°ç5˜ß¯rÖ, 1)J¤êABíî= d íl, Bb>áBíNû`¤ "/±²=, ÊFNûísu~2, .cÇó7BçXû ˝í ‰e…, "`¤f~í`çG, ¢bÊó¤çÞ®šíø…, ?UDÞ¿>â61J 5Ñ\š `¤JAâÇ[º.[óíj÷=Bíû˝G, ,u_v/ŸË#8‡D Nû, É-çÞ1q™TËÑçÞO; "`¤6ÊBböÅív`, .‘<íBb }®Þº%ð, 1/É-BbÊ{“5ÕíÞº ÊBí-ñ2, "`¤uøP,uÞ ®ñ, .}éçÞ>§ƒ9F>íç6, ?DÊ`¤íNû5-dû˝, Bg)}í•/ « wŸ, >á¨tãºn`¤ 0`¤ Ï£2`¤, ú¤dT|^~í<c, F bíNû, U…d?›ê¾ y6, >áõðf~íçÅÏN ÛïS ‰ †> D£Y ÏÀÃ, ÊB` Xƒ@½v, Fb,uÏ-í6ŒB, TXB.ý<c£õ¶, öí'ááFb °¢íÏn D ÅAý ŠT lT ˜.d, 'òEû˝Fsí¬˙2, ?DFbAóT, D Fbø–Uı, éû˝Dõðí¬˙rÖ QO, B;>áB°çÞ®2íߤ áá×ç혬i, áá5,uÊB`X•~í v`´-B, 1/´d_Gøš÷=Bí{“, 5´Bø–°z, Bn?DÊ×ù5(A ôˇì'ª, ß‚ËR¬,û˝F ááôÙ Ï| ØÌ Ùëj "Ô- ÙC] v.
(10) ðå Ù2 ápg s?Ê, ×çÞº5bí´-, éB¥û¬í'Ç-, 5bø– |Yí%ð, uBø2ä|1ßí[ |(, B;>áBÞ·2|½bíðA, ~~ yy, áá5b,uXMB, éB1B;1 íÍ, 6áá5b,u:Kí T, éB.Ûb>ç‘í98, ªJ-Ìê˛íêAç“, ?Dç5bíoä, Böí'« áácc __, çBƒð*7ív`, ,uªJ*5 b™,>§ƒðêíÅÊ, 'òEªJø_1Åíðê ¥¹díêA, uBÞ·2½bíøÜ, cø¤d.#{%6Œ¬BíAb. vi.
(11) Contents ` . . . . . . . . . . . . . . . . . . . . . . . . . . . . . . . . . . . . . .. i. Abstract . . . . . . . . . . . . . . . . . . . . . . . . . . . . . . . . . . .. iii. Acknowledgement . . . . . . . . . . . . . . . . . . . . . . . . . . . . .. v. Contents . . . . . . . . . . . . . . . . . . . . . . . . . . . . . . . . . . .. vii. List of Tables . . . . . . . . . . . . . . . . . . . . . . . . . . . . . . . .. ix. List of Figures . . . . . . . . . . . . . . . . . . . . . . . . . . . . . . .. xi. 1 Introduction. 1. 2 An UWB 3-dB Directional Coupler. 5. 2.1. Introduction . . . . . . . . . . . . . . . . . . . . . . . . . . . . . .. 6. 2.2. Theory . . . . . . . . . . . . . . . . . . . . . . . . . . . . . . . . .. 8. 2.2.1. Single-Section Directional Coupler . . . . . . . . . . . .. 8. 2.2.2. Multisection Directional Couplers . . . . . . . . . . . .. 15. 2.3. Analysis of the Vertically Installed Planar (VIP) structure. 17. 2.4. Design Procedure and Realization . . . . . . . . . . . . . . . .. 19. 2.4.1. Section 1 and Section 5 (Coupling= 15.92dB) . . . . .. 21. 2.4.2. Section 2 and Section 4 (Coupling= 7.54dB) . . . . . .. 23. vii.
(12) 2.4.3. Section 3 (Coupling= 0.82dB) . . . . . . . . . . . . . . .. 26. 2.4.4. The total cascaded circuit . . . . . . . . . . . . . . . . .. 30. 2.5. Compensated VIP structure . . . . . . . . . . . . . . . . . . . .. 32. 2.6. Measurement . . . . . . . . . . . . . . . . . . . . . . . . . . . . .. 38. 3 UWB Subharmonic Mixers. 43. 3.1. Introduction . . . . . . . . . . . . . . . . . . . . . . . . . . . . . .. 44. 3.2. Theory of a Diode Mixer . . . . . . . . . . . . . . . . . . . . . .. 45. 3.3. The Proposed UWB Subharmonic Quadrature-IF Mixer . .. 47. 3.3.1. Introduction . . . . . . . . . . . . . . . . . . . . . . . . . .. 47. 3.3.2. Circuit Realization and Measurements . . . . . . . . .. 49. The Proposed UWB Subharmonic Image Rejection Mixer .. 52. 3.4.1. Introduction . . . . . . . . . . . . . . . . . . . . . . . . . .. 52. 3.4.2. Circuit Realization and Measurements . . . . . . . . .. 56. 3.4. 4 Conclusion. 63. viii.
(13) List of Tables. 2.1. Table of parameters of symmetrical TEM coupled transmission line directional couplers . . . . . . . . . . . . . . . . . . . . . . . . . . .. 2.2. Table of parameters of five-section symmetrical TEM directional couplers . . . . . . . . . . . . . . . . . . . . . . . . . . . . . . . . . . .. 2.3. 20. 21. Physical dimensions of proposed five -section compensated directional coupler . . . . . . . . . . . . . . . . . . . . . . . . . . . . . .. ix. 40.
(14) x.
(15) List of Figures. 2.1. A single-section directional coupler . . . . . . . . . . . . . . . . . .. 9. 2.2. A directional coupler excited by even-mode sources . . . . . . . . .. 9. 2.3. A directional coupler excited by odd-mode sources . . . . . . . . . .. 10. 2.4. The simplified even-mode equivalent circuit . . . . . . . . . . . . . .. 10. 2.5. The simplified odd-mode equivalent circuit . . . . . . . . . . . . . .. 11. 2.6. Typical variation of coupling in a single section TEM coupler . . . .. 14. 2.7. An N-section asymmetrical parallel-coupled multisection directional coupler . . . . . . . . . . . . . . . . . . . . . . . . . . . . . . . . . .. 2.8. 15. An N-section symmetrical parallel-coupled multisection directional coupler . . . . . . . . . . . . . . . . . . . . . . . . . . . . . . . . . .. 16. Structure of a VIP directional coupler . . . . . . . . . . . . . . . . .. 18. 2.10 (a)Even- and (b)odd-mode equivalent circuits of VIP structure . . .. 18. 2.11 A five-section symmetrical coupler . . . . . . . . . . . . . . . . . . .. 22. 2.9. 2.12 (a)An ideal five-section symmetrical coupler and (b)its ideal response 22 2.13 A conventional parallel coupled line coupler . . . . . . . . . . . . .. 23. 2.14 Simulated results of section 1 and section 5 . . . . . . . . . . . . . .. 24. 2.15 Cross-sectional view of the type-I VIP coupler . . . . . . . . . . . .. 24. xi.
(16) 2.16 (a)Even- and (b)odd-mode equivalent circuits of the type-I VIP coupler 25 2.17 Even- and odd-mode characteristic impedances versus VIP metal height (Hmetal ) of the type-I VIP coupler with G = 28 mils . . . . .. 26. 2.18 Simulated results of section 2 and section 4 . . . . . . . . . . . . . .. 27. 2.19 Cross-sectional view of the type-II VIP coupler . . . . . . . . . . . .. 28. 2.20 (a)Even- and (b)odd-mode equivalent circuits of the type-II VIP coupler . . . . . . . . . . . . . . . . . . . . . . . . . . . . . . . . . . . .. 28. 2.21 Even- and odd-mode characteristic impedances versus Wg and Hmetal of the type-II VIP coupler . . . . . . . . . . . . . . . . . . . . . . .. 29. 2.22 Simulated results of section 3 . . . . . . . . . . . . . . . . . . . . .. 30. 2.23 Total cascaded circuit in Microwave Office . . . . . . . . . . . . . .. 31. 2.24 Simulated results of total cascaded circuit in Microwave Office . . .. 31. 2.25 3-D structure of the five-section directional coupler . . . . . . . . .. 32. 2.26 Simulated results of total cascaded circuit in HFSS . . . . . . . . .. 33. 2.27 A compensated VIP coupler . . . . . . . . . . . . . . . . . . . . . .. 34. 2.28 Top view of compensated VIP coupler . . . . . . . . . . . . . . . .. 35. 2.29 (a) Top and (b) cross-sectional view of modified compensated VIP coupler . . . . . . . . . . . . . . . . . . . . . . . . . . . . . . . . . .. 35. 2.30 Coupling (S31 ) of compensated structure with (a) L = 20mil and (b) W = 20mil . . . . . . . . . . . . . . . . . . . . . . . . . . . . . . . .. 36. 2.31 Return loss (S11 ) of compensated structure with (a)L = 20mil and (b) W = 20mil . . . . . . . . . . . . . . . . . . . . . . . . . . . . . xii. 37.
(17) 2.32 3-D structure of the five-section compensated directional coupler . .. 38. 2.33 Simulated results of the five-section compensated directional coupler in HFSS . . . . . . . . . . . . . . . . . . . . . . . . . . . . . . . . .. 39. 2.34 Photograph of the fabricated five-section 3-dB directional coupler .. 39. 2.35 Measured responses of the proposed hybrid . . . . . . . . . . . . . .. 40. 2.36 Compare between measured and simulated responses . . . . . . . .. 41. 2.37 Measured and simulate amplitude errors of the proposed directional coupler . . . . . . . . . . . . . . . . . . . . . . . . . . . . . . . . . .. 41. 2.38 Measured and simulate phase errors of the proposed directional coupler 42 3.1. Topology of subharmonic IRM . . . . . . . . . . . . . . . . . . . . .. 3.2. (a) I-V curve of a Schottky diode (b) transconductance waveform of Schottky diode . . . . . . . . . . . . . . . . . . . . . . . . . . . . .. 3.3. 45. 46. (a)Topology of UWB subharmonic quadrature-IF mixer (b)Diode current configuration . . . . . . . . . . . . . . . . . . . . . . . . . .. 48. 3.4. Photograph of the proposed UWB subharmonic quadrature-IF mixer. 50. 3.5. UWB subharmonic quadrature-IF mixer circuit configuration . . . .. 50. 3.6. Time domain wave form of quadrature-IF signal . . . . . . . . . . .. 51. 3.7. Conversion loss of quadrature-IF mixer . . . . . . . . . . . . . . . .. 52. 3.8. I/Q amplitude deviation of quadrature-IF mixer . . . . . . . . . . .. 53. 3.9. Quadrature phase deviation of quadrature-IF mixer . . . . . . . . .. 53. 3.10 (a) Topology of UWB subharmonic IRM (b) Diode current configuration . . . . . . . . . . . . . . . . . . . . . . . . . . . . . . . . . . xiii. 54.
(18) 3.11 Photograph of the proposed UWB subharmonic IRM . . . . . . . .. 57. 3.12 UWB subharmonic IRM circuit configuration . . . . . . . . . . . .. 58. 3.13 Conversion loss versus local power of IRM . . . . . . . . . . . . . .. 58. 3.14 Conversion loss versus RF frequency of IRM . . . . . . . . . . . . .. 59. 3.15 Image rejection ratio versus RF frequency of IRM . . . . . . . . . .. 60. 3.16 Conversion loss versus IF frequency of IRM. . . . . . . . . . . . . .. 60. 3.17 Image rejection ratio versus IF frequency of IRM . . . . . . . . . .. 61. 3.18 Isolation of LO to RF versus RF frequency of IRM . . . . . . . . .. 61. xiv.
(19) Chapter 1 Introduction. 1.
(20) Ultra-wideband (UWB) has received a lot of attentions in the wireless communication applications. It was first conceived in the 1960s and used for radar, sensing, and military communications in the past 20 years. The FCC opened 3.1-10.6 GHz available for UWB applications in 2002. UWB systems are focused on providing a low power, low cost, and wideband performance in a short distance. UWB systems can often encompass multiple gigaHertz of bandwidth, which poses some interesting problems to the system engineer. One of the most important is the need to reject the image frequency to avoid corruption of data or noise problems. The techniques used to realize different circuit components in a UWB transceiver are quite different from those proposed in narrow bandwidth radio frequency technology. One of the key elements in a UWB transceiver is the up- and down-conversion mixer. It is of interest to find a suitable mixer topology that can achieve good wideband performance in UWB systems. A wideband, large power, and stable local oscillator is expensive and hard to obtain. Thus, utilizing a subharmonic mixer to mix RF and LO signal becomes a better choice due to its halved bandwidth. There are two general techniques for image rejection. The first one is a preselected band pass filter, which is used to select the desired RF signal and reject the image one. The amount of image attenuation is strongly depends on the BPF design. If the IF frequency is quite small compared to LO and RF frequency, design a suitable BPF will be a hard task to the system designer. For broadband system such as UWB system, it is very difficult to built an IRM using filter. The second image rejection technique is known as phase-type. In this method 2.
(21) two mixing path with quadrature phase is provided and the properties of in phase and quadrature paths is exploited to attenuate the image. There is another benefit in this configuration such as improving the linearity by increasing the IP3 in comparison to a single mixer. A UWB subharmonic image rejection mixer (IRM) is proposed in this thesis, which the operation frequency is about 3-13.5GHz, totally covered the UWB bandwidth. The most common topology of subharmonic mixer was reported in [1] [2]. Although good performances were achieved, the topology mentioned above all need a Wilkinson power divider, two Anti Parallel Diode Pairs (APDP), a RF directional coupler, and an IF directional coupler. The subharmonic mixer proposed here only needs two diodes, a RF and IF directional coupler. However, the RF/LO quadrature hybrid has to be ultra-wideband. In this thesis, first, we will introduce the design procedure of the ultra-broadband RF/LO quadrature hybrid. Then improvement methods for return loss and isolation will be discussed. Finally, the proposed topology of UWB subharmonic mixer will be reported.. 3.
(22) 4.
(23) Chapter 2 An UWB 3-dB Directional Coupler. 5.
(24) 2.1. Introduction. A 3-dB quadrature coupler is an important and fundamental component in communication system. Nowadays, 3-dB quadrature couplers are widely used in many microwave circuits, such as low noise amplifiers, phase shifters, balanced mixers. Branch line coupler is an easy way of realizing the 3-dB quadrature coupler, but this method requires more space on the print circuit board and is very narrowband. A conventional coupler which consists of two parallel microstrip coupled lines is another method of 3-dB quadrature coupler realization. This method solve the problem of large area. However, it still has the trouble with the narrow gap between the two strips, due to its inherent weak coupling nature. By properly choosing the even- and odd-mode impedances of the two identical microstrip coupled lines as shown in Fig. 2.1, a four-port directional coupler can be obtained. Because of the coupling taking place in the backward direction, the coupler is also called a backward-wave directional coupler. The coupling value varies with frequency because the electrical length of the coupled line varies with respect to frequency. Thus, the varying coupling value causes some serious problems when we want to use a single section coupler in wide-band applications. A wider bandwidth can be achieved by multisection directional couplers. There was a complete analysis [3] for multisection directional couplers and the design had tabled into even- and odd-mode impedance for each section. There are other papers [4] [5] mentioned that the ultra-wideband performance can also be achieved by using multisection nonuniform cascaded couplers, but the length of nonuniform 6.
(25) multisection coupler is much longer than the uniform one. Besides, nonuniform couplers have some problems in complicated analysis. The extremely tight coupling at the center portion of the coupler also limits the practical implementation. Either uniform or nonuniform couplers have the problem with tight coupling, several methods have been proposed to solve the coupling problem in the literatures. One method is to use interdigital layout to achieve the tight coupling [6] [7]. The coupling value may higher than conventional coupled line, but still difficult to implement a coupler tighter than -3dB. Another approach is re-entrant structure [8] [9] [10], it can achieve tight coupling but hard to fabricate. Tandem couplers [11] might realize the extremely tight coupling section for the multisection directional couplers. Although this method is easy to implement, but the tandem couplers take large circuit area and have strong junction discontinuity effect. Vertically installed planar (VIP) structure is a method of solving the tight coupling problem. It was first proposed in [12] [13]. The VIP structure is composed of two substrates, the horizontal main substrate and the vertical (VIP) substrate which vertically installed on the horizontal one. The structure takes the advantages of easy fabrication, minimum layout area and low cost. This chapter is organized as follow. First the theory of coupler is discussed. Then multisection coupler and VIP structure are introduced. The design procedure of UWB multisection directional coupler, which utilizes VIP structure, is presented next. Finally, a compensating structure of VIP multisection directional coupler for return loss improvement is reported. 7.
(26) 2.2. Theory. Because we want to fabricate a ultra-broadband directional coupler suitable for UWB subharmonic mixer, the coupler must be operated over broader than 3-10GHz. According to [3], we must have a five-section TEM mode couplers, then the passband ±0.45dB ripple level and frequency bandwidth 1.5-13.5GHz can be realized. The 1.5 GHz low side frequency is due to the requirement of subharmonic LO.. 2.2.1. Single-Section Directional Coupler. A directional coupler has four ports, which are labeled as ”input,” ”direct,” ”coupled,” and ”isolated.” Three important parameters that characterize a directional coupler are its coupling and directivity, defined as below:. Coupling(dB) = 10 log. P1 P3. Directivity(dB) = 10 log Isolation(dB) = 10 log. P3 P4. P1 P4. (2.1). (2.2) (2.3). where P1 is the power input at port 1, P3 and P4 are the power output at port 3 and port 4, respectively. In an ideal case, there is no power transmitted out at isolation port; but in practice, a small amount of power is always coupled to this port. The schematic of a four port directional coupler is shown in Fig. 2.1. Since the coupler is a symmetrical structure, we can analyze the circuit by utilizing even- and odd-mode excitation. Fig. 2.2 and Fig. 2.3 are figures of the even- and odd-mode excitation, respectively. 8.
(27) Input port. Z0. 1. 2. Z0. Direct port. Z0o, Z0e Coupled port. Z0. 3. 4. Z0. Input port. Z0. 1. 2. Z0. 4 2. Z0. + 1V Coupled port. Isolated port. Direct port. Z0o, Z0e Z0 Z0. 3 1. Z0. Isolated port. Electric wall (short circuit) + 1V + 1V -. Z0 2.1: Z0 Z0o directional 3 A single-section 4 coupler Figure. Z0 1V ++ 1V -. 1. 2. Z0. Electric wall (short circuit) Z0. 3. Z0. 1. Z0o. 4. Z0. 2. Z0. Magnetic wall (open circuit). + 1V 1V +. Z0. 3. Z0e. 4. Z0. 1 2 Z0 Z0 Figure 2.2: A directional coupler excited by even-mode sources Magnetic wall (open circuit). + 1V -. Z0. 3. Z0e 9. 4. Z0.
(28) Z0o, Z0e Z0. 3. Electric wall (short circuit) Coupled Z0 3 port Z0o 4. + 1V Z0. Z0. 1. 1 2. 4. Z0. Z0. 2. Z0. Isolated port. Z0. Electric wall (short circuit) Magnetic wall (open circuit) Z0. 3. + 1V -. Z0e. Z0. 43. Z0. Z0o. Z0. 4. Figure 2.3: A directional coupler excited by odd-mode sources. 2. Z0. 1V +. Z0. 1. Z0e. 2. Z0. θe Z0. 1. 2. Z0. Magnetic wall (open circuit) Figure 2.4: The simplified even-mode equivalent circuit 4 Z0 3 Z0 Z0e. + Because the single section directional coupler is a symmetry structure, we can 1V simplify the four-port network to a two-port network, as shown in Fig. 2.4 and Fig. 2.5. The ABCD matrices for the even- and odd-mode equivalent circuit are given by . . . . jZ0e sin θe Ae Be cos θe = Ce De jY0e sin θe cos θe and. . . . (2.4a). . jZ0o sin θo Ao Bo cos θo = Co Do jY0o sin θo cos θo 10. (2.4b).
(29) Z0. 3. + 1V -. Z0. 1. Z0o. 2. Z0. 4. Z0e. Z0. θo. Figure 2.5: The simplified odd-mode equivalent circuit. The following relationship is useful when conversing the ABCD parameters to scattering parameters. S11 =. A + B/Zo − CZo − D A + B/Zo + CZo + D. (2.5a). S12 =. 2(AD − BC) A + B/Zo + CZo + D. (2.5b). S21 =. 2 A + B/Zo + CZo + D. (2.5c). S22 =. −A + B/Zo − CZo + D A + B/Zo + CZo + D. (2.5d). thus, the scattering parameters of simplified even- and odd-mode equivalent circuits can be obtained. According to (2.5), and. S11e + S11o 2 S21e + S21o = 2 S21e − S11o = 2 S21e − S21o = 2. S11 =. (2.6a). S21. (2.6b). S31 S41. (2.6c) (2.6d). we can obtain the scattering parameters of the single section directional coupler. 11. Z0. 1.
(30) After computiong the scattering matrix, the return loss (S11 ) will be (Z0e 2 Z0o 2 − Zo 4 ) sin θe sin θo S11 = 2 (Z0 + Z0e 2 ) sin θe − 2jZ0 Z0e cos θe (Z0 2 + Z0o 2 ) sin θo − 2jZ0 Z0o cos θo j(Z0 3 Z0e − Z0 Z0e Z0o 2 ) cos θe sin θo + j(Z0 3 Z0o − Z0 Z0o Z0e 2 ) sin θe cos θo + 2 (Z0 + Z0e 2 ) sin θe − 2jZ0 Z0e cos θe (Z0 2 + Z0o 2 ) sin θo − 2jZ0 Z0o cos θo (2.7) For an ideal parallel TEM coupler, the return loss should be zero. We can see if θe = θo and Z0e Z0o = Z0 2. (2.8). then (2.7) will identical to zero. (2.8) is a well-known condition of ideal parallel TEM coupler. If (2.8) is satisfied, the scattering parameters of the four pout network are given by S11 = S22 = S33 = S44 = 0. S12 = S21 S13 = S31. S14 = S41 = S23 = S32 = 0 √ 1 − k2 = S34 = S43 = S21e = √ 1 − k 2 cos θ + j sin θ jk sin θ = S24 = S42 = S11e = √ 1 − k 2 cos θ + j sin θ. (2.9) (2.10) (2.11) (2.12). where S11e and S12e denote the reflection and transmission coefficients of the coupled lines for the case of even-mode excitation, and θ = βl denotes the electrical length of the coupler. Coupling coefficient k is given by k=. Z0e − Z0o Z0e + Z0o. (2.13). for the coupling is given as C dB (where C is positive quantity), then k is related to C as k = 10−C/20 12. (2.14).
(31) The amount of coupling between ports 1 and 3 varies with the electrical length θ, and the maximum occurs when. θ = βl =. π rads 2. (2.15). or l=. π λg = 2β 4. (2.16). Besides, frequency bandwidth B and frequency bandwidth ratio w are also important parameters of a directional coupler, which are defined as f2 f1. (2.17). w=. f2 − f 1 f0. (2.18). f0 =. f2 + f 1 2. (2.19). B=. where. is the center frequency of the coupler and f2 and f1 are the upper and lower frequencies when the coupling equals C + δ, as shown in Fig. 2.4. δ is the tolerance amount and can be arbitrarily specified. The frequency bandwidth ratio B and frequency bandwidth ratio w are related by B−1 B+1. (2.20). w 2 B= w 1− 2. (2.21). w=2 and. 1+. 13.
(32) Z0. port. 1. 2. Z0. port. 4. Z0. Isolated port. Z0o, Z0e. Coupling (dB). Coupled port. Z0. 3. C+δ C C-δ. f1. f0. f2. Frequency Figure 2.6: Typical variation of coupling in a single section TEM coupler From (2.13), we can write Z0e /Z0o in terms of coupling coefficient k as Z0e 1+k = Z0o 1−k. (2.22). further, combine this equation with (2.8), we can get r Z0e = Z0. 1+k 1−k. (2.23). 1−k 1+k. (2.24). and r Z0o = Z0. When we want to design a coupler with a given coupling coefficient k and characteristic impedance Z0 , we first determine the even- and odd-mode impedances using (2.23) and (2.24), respectively. The physical length l of the coupler is chosen 14.
(33) Port 1. Section1. Input port. Coupled port. Section2. Z0. 1. Z0. 3. Z0o1 Z0e1. i=1. Section3. Section2. Port 3. Z0oN Z0eN. Z0o3 Z0e3. Z0o2 Z0e2. Section1. 2. Z0. 4. Z0. Direct port. ………. ………. i=2. i=3 i=N. Isolated port. Figure 2.7: An N-section asymmetrical parallel-coupled multisection directional coupler. Z0. Z0o1 Z0e1. 1. Z0oN Z0eN ……… ………. l=. λg 4. Z0. 2. Direct port. (2.25). ……… ………. Input as port. Coupled where Z 3 wavelength 4 medium Z0 i=1 i = (N+1)/2 i=N λ0g is the of the TEM wave in the transmission line at Isolated port port. the design frequency f0 .. 2.2.2. Multisection Directional Couplers S. 11e A single-section directional coupler has the benefit of easy fabrication, but it is. 1. Z0e1. Z0eN. 2. hard to obtain an ultra-wideband performance. In order to achieve a near-constant ……… ………. Z0. i=1. i = (N+1)/2. i=N. Z0. coupling over a wider frequency bandwidth, a number of coupled sections must be cascaded [3]. Each section is quarter-wave long at the center frequency. Fig. 2.7 shows an N-section asymmetrical parallel-coupled directional coupler. A multisection coupler can be either symmetrical or asymmetrical. The term symmetrical means that a coupler has end-to-end symmetry and employs an odd number of 15.
(34) Coupled port. Z0. 3. i=1. i=2. ………. i=3. 4. i=N. Input port. Z0. Z0o1 Z0e1. 1. ……… ………. Z0. 3. i=1. Z0oN Z0eN. 2. Z0. i=N. 4. Z0. Direct port. ……… ………. Coupled port. Isolated port. Z0. i = (N+1)/2. Isolated port. Figure 2.8: An N-section symmetrical parallel-coupled multisection directional coupler S11e 1. Z0e1. Z0eN. 2. sections. The ith section will be……… identical to the N + 1 − ith section in an N-section ………. Z0. i=1. i = (N+1)/2. i=N. Z0. symmetrical coupler as shown in Fig. 2.8. The coupler is referred to as an asymmetrical directional coupler if it does not have the end-to-end symmetry (Fig. 2.7). The main difference between symmetrical and asymmetrical coupler is the phase property. For a symmetrical directional coupler, the signal coupled to the coupled port has 90 degree phase difference with the signal coupled to the direct port, i.e. ∠S31 = ∠S21 + 90o . The phase relationship has a significant property that it is independent of frequency. Due to this property, applications for which symmetrical couplers are best suited including multiplexers, directional filters, balanced mixers, phase shifters, diplexers, and others to which the 90 degree difference property is essential. A additional advantage of symmetrical couplers is that the strongest coupling section is in the center and not at one end, so that it becomes less difficult to connect to all four ports. The asymmetrical couplers do not show the phase property of symmetrical couplers and are generally used where the couplers are designed to obtain broadband power division only. Other typical advantages may 16.
(35) include higher isolation and better input VSWR. One of the major limitations of a multisection coupler is that the coupling of the center section is much tighter than the overall coupling. This can create fabrication problems in microstrip technology where it is difficult to achieve tight coupling. Further, different phase velocities of even- and odd-mode equivalent circuits will degrade the coupler performance. We will use the VIP structure to solve the mentioned problems.. 2.3. Analysis of the Vertically Installed Planar (VIP) structure. A VIP circuit consists of broadside coupled lines vertically installed on a main substrate. A typical directional coupler using a VIP structure is shown in Fig. 2.9. The VIP substrate is installed on the main substrates. The metal patterns with coupled lines are made on both sides of the VIP substrate. The gray portions in Fig. 2.10 are transmission lines. Through conventional parallel coupled line, it is not easy to have strong coupling between two adjacent microstrip lines. On the other hand, in the VIP configuration, the vertical substrate contributes to a strong coupling. We can assume that the propagation is in the quasi-TEM mode, so that the capacitance between signal line and ground is the most important quantity. The effective dielectric constant and the characteristic impedance are computed as. e = 17. C C0. (2.26).
(36) 4 4. 3. 4. 2. 3. 2. 3. 2. 4. 2. 3. 1. 1. 1. 1 (a) VIP structure. (b) Top view. (a) VIP structure. (c) Side view. (b) Top view. (c) Side view. Figure 2.9: Structure of a VIP directional coupler Magnetic wall. Electric wall. 2Cb Ca. Ca. (a). (b). Figure 2.10: (a)Even- and (b)odd-mode equivalent circuits of VIP structure. Z0 = √. 1 e cC0. (2.27). where c is the light velocity in the free space, and C and C0 are the capacitance between the signal line and ground with and without dielectric substrate, respectively. Now we analyze the VIP structure with the even- and odd-mode equivalent circuits, as shown in Fig. 2.10. The magnetic wall (even-mode) and electric wall (odd-mode) are located at the symmetry planes of the VIP substrate, respectively. 18.
(37) The magnetic wall acts like an open circuit and the electric wall acts like an short circuit. The even-mode equivalent capacitor contains only Ca , but the odd-mode equivalent capacitor contains Ca and 2Cb . Because the distance (4mil) between the symmetry plane and the conductor is very small, 2Cb becomes the major term by its large value. The odd-mode equivalent capacitance, which contains 2Cb , is much larger than the even-mode equivalent capacitance. Thus, by (2.26) and (2.27), the odd-mode characteristic impedance (Z0o ) will be much smaller than the even-mode characteristic impedance (Z0e ). We can adjust the heights of the metal patterns on the VIP substrate to fit the desired even- and odd-mode characteristic impedance. In the odd-mode excitation, due to the major capacitance 2Cb , the electric filed is mostly confined in the VIP substrate (r = 3.38). In the even-mode excitation, most of the electric field is in the air (r = 1). Therefore, the VIP structure has inherently different even- and odd-mode phase velocities. The phase velocity of oddmode is slower than the even-mode, this will cause poor isolation and directivity. We will put the dielectric blocks, which are the same materials as the main and VIP substrate, to solve the problem. After adding the dielectric blocks, the phase velocity of even- and odd-mode can be nearly equal.. 2.4. Design Procedure and Realization. We want to design a symmetrical multisection TEM coupler with the following specifications:. Center frequency = 7.5GHz 19.
(38) δ 0.05 0.10 0.15 0.20 0.25 0.30 0.35 0.40 0.45 0.50 0.60 0.70 0.80 0.90 1.00. Z1 1.05792 1.07851 1.09451 1.10921 1.12314 1.13659 1.13973 1.16266 1.17547 1.18822 1.21370 1.23941 1.26555 1.29235 1.31998. Z2 1.32624 1.37268 1.40890 1.44029 1.46883 1.49551 1.52091 1.54541 1.56926 1.59265 1.63864 1.68425 1.73013 1.77678 1.82466. Z3 3.81243 3.97615 4.10191 4.21023 4.30864 4.40089 4.48917 4.57491 4.65912 4.74253 4.90924 5.07867 5.25363 5.43655 5.62978. w 1.20488 1.32559 1.39889 1.45184 1.49333 1.52744 1.55639 1.58152 1.60371 1.62357 1.65791 1.68691 1.71196 1.73402 1.75370. B 4.03071 4.93114 5.65437 6.29714 6.89474 7.46462 8.01698 8.55845 9.09367 9.62609 10.69292 11.77568 12.88720 14.03860 15.24047. Table 2.1: Table of parameters of symmetrical TEM coupled transmission line directional couplers Mean coupling = 3dB. Maximal ripple level = ±0.45dB. Operating frequency = 1.5 − 13.5GHz. Frequency bandwidth ratio = 9. Cristal and Young had generated design tables for equal-ripple symmetrical couplers [3], the design table is reproduced in Table 2.1. We use the Table 2.1. to design a symmetrical coupler. It can be found that a 3-dB, five-section coupler designed for a ripple level of ±0.45dB exhibits a frequency bandwidth ratio B = 9.09367. Because this figure of B is very close to the specified value of B = 9, it is sufficient to employ a five-section coupler to achieved the desired specifications. 20.
(39) Z0e (Ω) Z0o (Ω) k Coupling(dB) δ(dB) w B. Section 1,5 58.77 42.54 0.16 15.92. Section2,4 78.46 31.86 0.42 7.54 0.45 1.60 9.1. Section3 232.96 10.73 0.91 0.82. Table 2.2: Table of parameters of five-section symmetrical TEM directional couplers The parameters in Table 2.1 are the normalized values of even-mode characteristic impedance. We can multiply the parameters by 50 to obtain the actual even-mode characteristic impedance. After knowing the Z0e of each section, Z0o , k and coupling in dB can be found by (2.8), (2.13) and (2.14). The values of Z0e , Z0o , and coupling for the desired five-section symmetrical coupler are given in Table 2.2. The figure of five-section symmetrical coupler is shown in Fig. 2.11. Fig. 2.12 is an ideal five-section symetrical coupler and its ideal response, which is simulated by Microwave Office. Note that each section is quarter-wave long at the center frequency. Section 1 and section 5 can be fabricated by parallel coupled line coupler. But if section 2 and section 3 are implemented by the same method, the gap between the coupled lines will be 1.78mil and 0.066mil, respectively, which are impractical designs. VIP structure may be a better choice to implement the tight coupling section.. 2.4.1. Section 1 and Section 5 (Coupling= 15.92dB). From Table 2.2, the section 1 and section 5 are loosly coupled sections. The conventional parallel coupled line coupler is suitable for realizing these two sections. 21.
(40) Input port. Direct port. Coupled port. Section1. Section2. Section3. Section4. Isolated port. Section5. Figure 2.11: A five-section symmetrical coupler Input port. Coupled port. Z0. 1. Z0. 3. Z0o1 Z0e1. i=1. Z0oN Z0eN. Z0o3 Z0e3. Z0o2 Z0e2. 2. Z0. 4. Z0. Direct port. ………. ………. i=2. i=3 i=N. Isolated port. (a). 0Z. 0. 1. Z0o1 Z0e1. S11. Z0 -40 (dB). S41. ……… ………. -20. Coupled port. S31. S21. 3. i=1. Z0oN Z0eN. 2. Z0. i=N. 4. Z0. Direct port. ……… ………. Input port. i = (N+1)/2. Isolated port. -60 -80 -100. S11e 1 2.5 Z0. Z0e1. 5. ………. i=1. 7.5 Frequency (GHz) i = (N+1)/2. 10. Z0eN. ………. -120. 12.5. i=N. 215 Z0. (b). Figure 2.12: (a)An ideal five-section symmetrical coupler and (b)its ideal response. 22.
(41) Ca. Ca. (a). (b). length. gap. width. Figure 2.13: A conventional parallel coupled line coupler The parallel coupled line coupler is shown in Fig. 2.13. The specifications of section 1 and section 5 are Z0e = 58.77Ω, Z0o = 42.54Ω,and Coupling = 15.92dB. Roughly calculating the gap, width and the length of coupled line, we obtained the gap = 15mil, width = 40mil, length = 233mil. By using the EM simulator such as Ansoft HFSS to simulate circuit, the simulated results of scattering parameters is shown in Fig. 2.14. The Coupling (S31 ) in Fig. 2.14 is 15.53dB, which is very close to the specified value, and the center frequency is indeed at 7.5GHz.. 2.4.2. Section 2 and Section 4 (Coupling= 7.54dB). The Coupling of section 2 and section 4 should be 7.54dB, this cannot be implemented by the conventional parallel coupled line coupler due to physical limitation. There are two different types of VIP coupler were proposed in [14]. A type-I VIP coupler is shown in Fig. 2.15. There are four metal strips of which two are on the main substrate and the other two are on the VIP substrate. The strips 23.
(42) SS2121. S11. S31. S41. 10 0. (dB). -10 -20 -30 -40 -50 2.5. 5. 7.5. 10. 12.5. 15. Frequency (GHz). Figure 2.14: Simulated results of section 1 and section 5. S21. S11. S31. S41. 10 0. (dB). -10 -20. WVIP. -30. Hmetal. H VIP. -40 -50 2.5. 5. Metal. 7.5. 10. 12.5. 15. Frequency (GHz) Dielectric substrate. W G. S21. S11 Magnetic wall. S31 Electric wall. S41. Figure 2.15: Cross-sectional view of the type-I VIP coupler 0 -5. (dB). -10 -15. 24 -20 -25. Ca. 2Cb Ca. Ca. Cc. Cc. -30 2.5(a). 5. 7.5. 10. (b). 12.5. 15.
(43) H VIP. Hmetal. Metal. Dielectric substrate. W G. Magnetic wall. Electric wall. 2Cb Ca. Ca. Ca. Cc. Cc. (a). (b). WVIP. Figure 2.16: (a)Even- and (b)odd-mode equivalent circuits of the type-I VIP coupler. Hmetal. H VIP. on the main and VIP substrates are connected at two ends of the coupler. The Metal. performance of the coupler is improved by adding dielectric blocks at both sides of the VIP substrate, which use the same material as the main substrateDielectric and VIP substrate. substrate. This coupler can implement a coupler with coupling from moderate to WG. tight coupling.. Wgnd. TheMagnetic even-wall and odd-mode equivalent circuits of the type-I VIP coupler is shown Electric wall in Fig. 2.16. It shows that the total equivalent capacitance of each equivalent circuit is the combination of every capacitance shown in the figure. This means that three degrees of freedom, which are the VIP metal height, the width of the strips on the 2C2C b b Ca. C. a Ca the gap width on the main Ca main substrate, and substrate, are available to choose. the even- and odd-mode characteristic impedances. The total width of the coupler (a). (b). has to be chosen carefully so that the junctions connecting to other sections can be laid out with minimal discontinuity. The even- and odd-mode characteristic impedances with respect to the VIP 25.
(44) Z0e. }Z. 0o. Figure 2.17: Even- and odd-mode characteristic impedances versus VIP metal height (Hmetal ) of the type-I VIP coupler with G = 28 mils metal height and the width of the strips on the main substrate are extracted by the EM simulator Ansoft HFSS. Fig. 2.17 depicts the extracted data, in which the gap Z0e. width between two strips on the main substrate 28 mils. As given in Fig. 2.17, Hmetal = 7mil, W = 15mil, G = 28mil are chosen for the case if Z0e = 78.46Ω and Z0o = 31.86Ω. The simulated results of scattering Z0o. parameters is shown in Fig. 2.18. The Coupling (S31 ) is 7.7dB at 7.5GHz, which is very close to the ideal value 7.54dB.. 2.4.3. Section 3 (Coupling= 0.82dB). The type-I VIP coupler can not implement the extremely tight-coupled center section even when W equals 0. To achieve a coupling value as tight as 0.8 dB, the type-II VIP coupler is proposed in [14], as shown in Fig. 2.19. The ground plane 26.
(45) -50 2.5. 5. 7.5. 10. 12.5. 15. 12.5. 15. Frequency (GHz). S11. S21 S21. S31S31. S41. 10 0. (dB). -10 -20 -30 -40 -50 2.5. 5. 7.5. 10. Frequency (GHz). Figure 2.18: Simulated results of section 2 and section 4. of type-II VIP coupler to twoSmetal strips. Utilizing S21 substrate Schanges S11 in the main 31 41 0. this finite-extent ground plane, the VIP coupler can achieve a coupling tighter than -5 0.8 dB. Again, a dielectric block as the type-I is used to compensate the modal. (dB). phase-10 velocities. -15. The type-II VIP coupler also has three degrees of freedom to choose the even-20. and odd-mode characteristic impedance such as the type-I VIP coupler. The even-25. and odd-mode equivalent circuits of the type-II VIP coupler are shown in Fig. 2.20. -30. The larger the gap between the two strips on the ground plane, the smaller the 2.5. 5. 7.5. 10. 12.5. 15. Frequency (GHz)impedance of even-mode can be equivalent capacitance. Thus, the characteristic. controlled by the Wg . In addition, the width of ground strips also has influence on Z0e . The metal height on the VIP substrate can affect the odd-mode characteristic 27.
(46) etal. Dielectric substrate. W G. 2Cb Ca Ca wall Magnetic. Ca Electric wall. Cc. Cc. (a). (b). WVIP. 2Cb Ca. Ca. Ca. Cc Hmetal. H VIP. Cc Metal. (a). (b). Dielectric substrate. WVIP. WG. Hmetal. H VIP. Wgnd. Metal. Figure 2.19: Cross-sectional view of the type-II VIP coupler. Magnetic wall. Electric wall. Dielectric substrate. WG Wgnd. 2C2C b b C Ca a Magnetic wall. Ca CaElectric wall. (a). (b). 2C2C b b C Ca a. Ca Ca. (a). (b). Figure 2.20: (a)Even- and (b)odd-mode equivalent circuits of the type-II VIP coupler. 28.
(47) Z0e. Z0o. Figure 2.21: Even- and odd-mode characteristic impedances versus Wg and Hmetal of the type-II VIP coupler. impedance. A large value of height of the metal on the VIP substrate will make itself and the electric plane forming a giant parallel plate capacitor. This means that the odd-mode characteristic impedance will significant decrease if the metal height becomes larger.. The even- and odd-mode characteristic impedances with respect to the Wg and Hmetal of the type-II VIP coupler are shown in Fig. 2.21. According to this figure, the Wg , Hmetal , and Wgnd are chosen to be 208mil, 60mil, and 16mil, respectively. The simulated result is shown in Fig. 2.22. The Coupling in Fig2.22 only has 0.02dB difference to the ideal value. 29.
(48) -50 2.5. 5. 7.5. 10. 12.5. 15. Frequency (GHz). S21. S11. S31. S41. 0 -5. (dB). -10 -15 -20 -25 -30 2.5. 5. 7.5. 10. 12.5. 15. Frequency (GHz). Figure 2.22: Simulated results of section 3. 2.4.4. The total cascaded circuit. After finishing the design of each section of the UWB multisection directional coupler, the scattering parameters of UWB multisection directional coupler are obtained by cascading each section, utilizing a circuit simulator Microwave Office. The total cascaded circuit in Microwave Office is shown in Fig. 2.23 and simulated results are shown in Fig2.24. The S21 and S31 shown in Fig. 2.24 are very close to the S21 and S31 of an ideal five-section directional coupler, as shown in Fig. 2.12. But the isolation and return loss of this cascaded circuit are quite different from the ideal value. Fig. 2.24 shows that the initial designs of each section are dependable. When each section cascaded one by one, the junction discontinuity must be taken into 30.
(49) (dB). -10. -20. -30 2.5. 5. 7.5. 10. 12.5. 15. Frequency (GHz). Figure 2.23: Total cascaded circuit in Microwave Office. S11. S21. SS3131. S41. 10. (dB). 0. S11. S21. S31. S41. 0. -10. -10. (dB). -20 -20. -30 -30. 2.5. 5. 7.5. 10. 12.5. 15. Frequency (GHz) -40. 2.5 results 5of total7.5 10 circuit12.5 15 Figure 2.24: Simulated cascaded in Microwave Office Frequency (GHz). 31.
(50) Figure 2.25: 3-D structure of the five-section directional coupler consideration. In order to get more accurate results, the whole five-section direcS 31. S21. tional coupler is simulated by an EM simulator Ansoft HFSS. The 3-D structure. (dB). of the five-section directional coupler is shown in Fig. 2.25, and simulated results S11 are shown in Fig. 2.26. Fig. 2.26(a) shows that the useful bandwidth of this mul-. tisection directional coupler is from 1.5 to 13.5GHz. The maximalS41amplitude error between the coupled port and direct port is about 1.2dB (ideal value is 0.9dB). The phase of the coupled and direct port has a 90 degree difference due to its symmetric nature. (a) Fig. 2.26 shows the performance degradation at high frequency. The main. reason for the degradation is from the junction discontinuity effect between each section, especially between section 3 and its neighbors. The return loss is -18dB at 10.75GHz, which is not good enough. A compensated VIP structure is useful for (degree). the return loss improvement.. 2.5. Compensated VIP structure. In order to improve the performance of a VIP coupler, a new planar compensation S21 S31 structure has been studied experimentally and theoretically in [15]. By only adding 32 (b).
(51) S21. (dB). S31. S11. S41. (degree). (a). S21. S31. (b). Figure 2.26: Simulated results of total cascaded circuit in HFSS. 33.
(52) 2. 4. 1. Metal. Dielectric substrate. 3. W1. Figure 2.27: A compensated VIP coupler. 4 2 some simple short microstrip stubs to the VIP coupler circuit, a better frequency Metal. response on the S parameters can be obtained. L L 1. Dielectric A configuration3 of the proposed compensated VIP directional coupler is illus1 substrate. trated in Fig. 2.27.. L Fig. 2.28 is just a top view of this device. The length L is 2. W chosen as a quarter wavelength at the center frequency. The compensation stubs 2. (W2 × L2 ) at the terminals of the coupling sections will influence mainly on the magnitude of the return loss and isolation, and the compensation stub in the center will contribute mainly to reduce the difference between the even and odd mode effective dielectric constants. A modified topology suitable for the multisection directional coupler is shown L. in Fig. 2.29. The center compensation stub is removed since the magnitude of the isolation and return loss are the major issue. W. Thesection results Preceding. of return loss (S11 ) and coupling (S31 ) with respectsection to the stub width succeeding. and length are simulated by Ansoft HFSS, as shown in Fig. 2.30 and 2.31. (a). 34. Metal.
(53) 1. 3. substrate. 2. 4. Metal. W1. 1. Dielectric substrate. 3. 4. 2 Metal W1 L1. L. 3. Dielectric substrate. 1. 4. 2 Metal. L2. L. L1. W2. 3. Dielectric substrate. 1 L2. W2. Figure 2.28: Top view of compensated VIP coupler. L W preceding section. L. succeeding section. (a). W Preceding section. succeeding section Metal (a) Dielectric substrate. (b). Metal. Figure 2.29: (a) Top and (b) cross-sectional view of modified compensated VIP Dielectric coupler substrate (b). 35.
(54) W=10mil. W=20mil. W=30mil. W=40mil. uncompensated. 0. (dB). -5. -10. -15 2.5. 5. 7.5. 10. 12.5. L=40mil. uncompensated. 15. Frequency (GHz) (a) L=10mil. L=20mil. L=30mil. 0. (dB). -5. -10. -15 2.5. 5. 7.5. 10. 12.5. 15. Frequency (GHz) (b). Figure 2.30: Coupling (S31 ) of compensated structure with (a) L = 20mil and (b) W = 20mil 36.
(55) W=10mil. W=20mil. W=30mil. W=40mil. uncompensated. 0 -10. (dB). -20 -30 -40 -50 -60 2.5. 5. 7.5. 10. 12.5. 15. Frequency (GHz) (a) L=10mil. L=20mil. L=30mil. L=40mil. uncompensated. 0 -10. (dB). -20 -30 -40 -50 -60 2.5. 5. 7.5. 10. 12.5. 15. Frequency (GHz) (b). Figure 2.31: Return loss (S11 ) of compensated structure with (a)L = 20mil and (b) W = 20mil 37.
(56) : compensated stubs. Figure 2.32: 3-D structure of the five-section compensated directional coupler Observing Fig. 2.30, the coupling maintains the same value, no matter how the S31. S21. width or length changes. Fig. 2.31 shows that the return loss varies with the width or length. These results show that the terminal stubs only affect the magnitude of S11 coupling. return loss and have no effect on the S41. After choosing W = 20mil and L = 20mil, which make return loss having the lowest value, we adopt the compensated section into the total cascaded circuit to implement the UWB multisection directional coupler. The 3-D structure of the compensated multisection coupler is shown in Fig. 2.32. Fig. 2.33 is the simulated results by utilizing Ansoft HFSS. Compare Fig. 2.33 with the previous result Fig. 2.26, the return loss has a significant improvement by at least 6dB at 7.5-12.5GHz. The benefits of the compensated structure are the return loss can be effective suppress and the coupling won’t be affected by adding the compensated stubs.. 2.6. Measurement. The photograph of the proposed compensated five-section directional coupler is shown in Fig. 2.34. The main substrate, VIP substrate, and dielectric blocks are 38.
(57) : compensated stubs. S31. S21. S11 S41. Figure 2.33: Simulated results of the five-section compensated directional coupler in HFSS. S11. S21. S31. 0 -20. (dB). -40 -60 -80 -100 -120 Figure 2.34: Photograph of the fabricated five-section 3-dB directional coupler 2.5. 5. 7.5. Frequency (GHz Measurement S31 S21 0 -2 -4. 39. -6 (dB). -8 -10 -12 -14 -16. Simulation S31. S21.
(58) S11. S21. S31. S41. 0 -10. (dB). -20 -30 -40 -50 -60 2.5. 5. 7.5. 10. 12.5. 15. Frequency (GHz) Measurement S31 S21. Simulation SFigure 2.35: SMeasured responses of the proposed hybrid 31 21. Section 1,5 2,4 3. Z0e 58.77 78.46 232.96. Z0o 42.54 31.86 10.73. W 40 15 NA units. G Hmetal 15 NA 28 7 NA 60 (mil). Wg NA NA 208. Wgnd NA NA 16. L 233 200 209. Table 2.3: Physical dimensions of proposed five -section compensated directional coupler. all Rogers RO4003 with a dielectric constant of 3.58 and thickness of 8, 20, and 2.5. in Figs. 2.35 560 mils, respectively. 7.5 10Depicted12.5 15 is the measured result which matches Frequency (GHz) well with the simulation result as shown in Fig. 2.36. The simulated and measured. amplitude and phase errors are shown in Fig. 2.37 and Fig. 2.38, respectively. Simulation. Measurement. The measured amplitude balances between port 3 (coupled port) and port 2 (direct port) is less than 2dB, and the phase difference is keeping near 90 degree over the designed frequency of 1.5-13.5 GHz. 40.
(59) (dB). -60 -80 -80 -100 -100 -120 2.5. 5. 2.5. 5. 7.5. 10. -120. (dB) (dB). 0 -2 0 -4 -2 -6 -4 -8 -6 -10 -8 -12 -10 -14 -12 -16 -14 -18 -16 -20 -18. Measurement S31 S21. S31. Measurement S31 S21. S31. 2.5. 5. 2.5. 5. Simulation. Frequency (GHz) 7.5 10 Frequency (GHz). S21. Simulation. 7.5. -20. S21. 10. 12.5. 15. Frequency (GHz) 7.5 10. 12.5. 15. (GHz) Figure 2.36: Compare betweenFrequency measured and simulated responses Simulation. Measurement. Simulation. Measurement. |S21|-|S|S 31|(dB) 21|-|S31|(dB). 10 8 10 6 8 4 6 2 4 0 2 -2 0 -4 -2 -6 -4 -8 -6 -10 -8 -10. 2.5. 5. 2.5. 5. 7.5. 10. 12.5. 15. Frequency (GHz) 7.5 10. 12.5. 15. Frequency (GHz) Simulation Measurement 200 Measured and simulate amplitude errors of the proposed directional Figure 2.37: Simulation Measurement coupler erence (degree) se difference (degree). 200. 100. 41 100 0 0.
(60) -4 -6 -8 -10. 2.5. 5. 7.5. 10. 12.5. 15. 12.5. 15. Frequency (GHz). Simulation. Measurement. Phase difference (degree). 200. 100. 0. -100. -200 2.5. 5. 7.5. 10. Frequency (GHz). Figure 2.38: Measured and simulate phase errors of the proposed directional coupler We restate the design procedure of UWB multisection directional coupler as follows. According to the design table listed in Table 2.1 or in [3], five-section coupler are needed to fulfill such specifications. The corresponding even- and oddmode characteristic impedances of each section are detailed in Table 2.2. For the first and fifth sections, use a circuit simulator to get physical dimensions. Then, use Fig. 2.21 to obtain the physical dimensions of section 3. Last, to design sections 2 and 4, designers should properly choose the width and distance of the strips on the main substrate by the aid of Fig. 2.17 to minimize the discontinuities between sections. Finally, the whole directional coupler performances are simulated by 3-D EM simulator Ansoft HFSS. The physical dimensions of each section are shown in Table 2.3.. 42.
(61) Chapter 3 UWB Subharmonic Mixers. 43.
(62) 3.1. Introduction. One of the benefits of designing a system for operation in the milimeter wave band is the capability to utilize large bandwidths for the transmission of high volumes of data. How to reject the image frequency to avoid noise and data corruption is the major issue for an UWB mixer. Whilst in a narrow band system a simple filter can be chosen to do this, it can be impossible in a wide band system. A way of avoiding this issue is to incorporate an image reject mixer that, with the use of directional couplers, can inherently reject the image signal. Image rejection mixers are becoming more important, since the requirement for cheaper and smaller are becoming a trend for every 3C products. There are additional benefits provided by IRM topology to the system designer. First, the use of two mixers in a balanced configuration increases the IP3 by 3dB from a single mixer, and thus improving the linearity and allowing higher input RF power for the same spurious response. This achieves better spurious free dynamic range in a receiver. Secondly, the system designer can choose to isolate either an upper or lower sideband simply by modifying which port of IF directional coupler is the output. Finally, the use of directional couplers to apply the signals to the mixers inherently gives good port return loss and improved isolations. The subharmonic mixer can be driven by a local source with half of the LO frequency. In fact, the RF signal is mixed with the second harmonic of the LO source. This characteristic is useful in the wideband application since the wideband local oscillator is hard to obtain. The most common topology of subharmonic image 44.
(63) LO. Wilkinson Power Divider. RF 90o Hybrid. RF. Subharmonic mixer. IF 90o Hybrid. IF. Figure 3.1: Topology of subharmonic IRM. LPF. HPF. rejection mixer was proposed in [1] [2]. The mixer consists of a Wilkinson power divider, two Anti Parallel Diode Pairs (APDP), a RF directional coupler and an IF ID1. directionRF coupler, as shown in Fig. 3.1. RF/LO IF1 A 90º The UWB quadrature-IF and image rejection mixer Hybrid LO subharmonic IF2proposed in B I. ID2'. HPF. LPF. this thesis can be fabricated without a Wilkinson D2power divider and only needs two diodes. It has the benefit of reducing the number of elements.. 3.2. (a) Theory of a Diode Mixer. VRF,A shows the I-V curve of a Schottky diode. When VLO,A applying a local oscillatFig. 3.2(a). ing (LO) signal on a diode, the signal would be rectified since only the positive cycle can turn on the diode. Hence, the transconductance waveform gLO (t) of Schottky ID1,RF. ID1,LO. diode is shown in Fig. 3.2(b). The Fourier expansion of gLO (t) can be expressed as. gLO (t) = VRF,B. ∞ X n=−∞. gn ejnωLO t. (3.1) VLO,B. 45. ID2,RF. ID2.LO.
(64) ID2,RF. ID2.LO. (b). I VLO(t) gLO(t). t. V. 1/fLO (a). (b). Figure 3.2: (a) I-V curve of a Schottky diode (b) transconductance waveform of Schottky diode. When RF signal is applied on the diode at the same time, due to the nonlinearity of the diode, the RF voltage across the diode will contain harmonic terms of the RF frequency. This RF voltage is shown as. VRF (t) =. ∞ X. Vm ejmωRF t. (3.2). m=−∞. thus, the diode current id can be expressed as. id (t) =. ∞ X. ∞ X. gn Vm ej(mωRF +nωLO )t. (3.3). m=−∞ n=−∞. id shown above contains all intermodulation products of the RF frequency and LO frequency. Fundamental mixing occurs when (m,n) equals (1,-1) or (-1,1). All other higher order products can be eliminated by a filter. 46.
(65) 3.3 3.3.1. The Proposed UWB Subharmonic QuadratureIF Mixer Introduction. The proposed topology of UWB subharmonic quadrature-IF mixer is shown in Fig. 3.3(a). The RF/LO 90o hybrid in Fig. 3.3(a) is identical to the UWB multisection directional coupler proposed in chapter 2. The RF and LO input signal are applied to the input port and isolated port in Fig. 2.11, respectively. RF signal is isolated with LO signal due to the property of a directional coupler. We analyze the proposed mixer by the relationships between diode current and LO, RF signal. Fig. 3.3(b) shows that the RF signal at point B, which is marked in Fig. 3.3(a), has a 90 degree phase delay to the RF signal at point A. Here, we only concerned the phase relationship and ignored the amplitude changing. LO signal at point A also has a 90 degree phase delay to the LO signal at point B. Thus, the diode current ID2,RF of RF signal has a 90 degree phase ahead of ID1,RF . The relation between ID2,RF and ID1,RF can be expressed as ID2,RF = (j)m ID1,RF. (3.4). also, ID1,LO and ID2,LO has the following relationship ID2,LO = (−j)n ID1,LO. (3.5). after combining the equations above, the total relation between ID1 and ID2 can be shown as ID2 = (j)m (−j)n ID1 47. (3.6).
(66) IF 90o Hybrid. LPF. HPF. IF. ID1. RF LO. RF/LO 90º Hybrid. A. IF1. B. IF2 LPF. ID2'. HPF. ID2. (a). VRF,A. VLO,A. ID1,RF. ID1,LO. VRF,B. VLO,B. ID2,RF. ID2.LO. (b). Figure 3.3: (a)Topology of UWB subharmonic quadrature-IF mixer (b)Diode current configuration. 48.
(67) the index m and n in the equations above represent the harmonics of RF and LO 0 means the diode current of reversing the direction of ID2 signal. ID2. 0 ID2 = −ID2 = −(j)m (−j)n ID1. (3.7). IF signal appears when (m,n) equals (1,-2) or (-1,2) in a subharmonic mixer. For (m,n) equals (1,-2) 0 ID2 = −j(−j)−2 ID1 = jID1. (3.8). 0 where ID1 has a phase delay of 90 degree compared to ID2 . On the other hand, for. (m,n) equals (-1,2) 0 ID2 = −(j)−1 (−j)2 ID1 = −jID1. (3.9). 0 ID2 has a phase delay of 90 degree compared to ID1 . The above property actually. performed a quadrature-IF mixer.. 3.3.2. Circuit Realization and Measurements. Fig. 3.4 and Fig. 3.5 show photograph and configuration of the proposed UWB mixer. The circuit is fabricated on RO4003 substrate with a dielectric constant of 3.58 and thickness of 20mil. The RF and IF frequency are chosen to be 3-13GHz and 60MHz, respectively. The total circuit consists of a UWB RF/LO directional coupler, two diodes, a low-pass filter, and a high-pass filer. UWB RF/LO directional coupler utilizes the multisection direction coupler discussed in chapter 2. LPF and HPF are realized by a simple conductor and capacitor. 49.
(68) Figure 3.4: Photograph of the proposed UWB subharmonic quadrature-IF mixer RF. IF. HPF LPF. RF/LO hybrid. Ground pad IF. LO. Figure 3.5: UWB subharmonic quadrature-IF mixer circuit configuration 50. RF. IF.
(69) O. − f RF. = 7GHz. 20°. Figure 3.6: Time domain wave form of quadrature-IF signal. f RF = 8GHz 90°. Applying sinusoidal waves into RF and LO port, a time-domain voltage waveform can be observed by an oscilloscope. Fig. 3.6 is the measured voltage waveform of proposed mixer under the condition of local power 10dBm, RF frequency 8GHz. Fig. 3.6 shows that the amplitude deviation is almost zero at this frequency and phase difference between two output ports is 90 degree. Fig. 3.7 shows the conversion loss of the quadrature-IF mixer. The conversion loss is lower than 15dB when local power is larger than 10dBm. The RF and IF frequency are given at 8GHz and 60MHz, respectively.. 51.
(70) 40. Conversion Loss (dB). 35. 30. 25. 20. 15. 10 0. 2. 4. 6. 8. 10. 12. 14. 16. Local Power (dBm). 26. Figure 3.7: Conversion loss of quadrature-IF mixer. 24 and Fig. 3.9 show the I/Q amplitude deviation and quadrature phase Fig. 3.8. Isolation (dB). deviation22of the quadrature-IF mixer. The amplitude deviation is less than ±3dB when RF20frequency is from 3-13GHz and IF is fixed at 60MHz. The local source power is set 18 around 10dBm.. 3.4 3.4.1. 16. The Proposed UWB Subharmonic Image Re14 jection Mixer 12. Introduction 2 4. 6. 8. 10. 12. 14. RF Frequency (GHz). The proposed topology of UWB subharmonic image rejection mixer (IRM) is shown in Fig. 3.10. This image rejection mixer is same as the quadrature-IF mixer discussed in the previous section except an additional IF hybrid. We utilize the same method to analyze the proposed mixer. 52.
(71) -60 -80 2. 4. 6. 8. 10. 12. 14. RF Frequency (GHz). 4. I/Q Amplitude Deviation (dB). 3 2 1 0 -1 -2 -3 2. 4. 6. 8. 10. 12. 14. RF Frequency (GHz). Figure 3.8: I/Q amplitude deviation of quadrature-IF mixer. 3.9 3.8. 60 40 20 0 -20 -40 -60 -80 2. 4. 6. 8. 10. 12. 14. RF Frequency (GHz). Figure 3.9: Quadrature phase deviation of quadrature-IF mixer. 4 3. n (dB). Quadrature Phase Deviation (dB). 80. 2. 53.
(72) HPF. LPF. ID1. RF/LO 90º Hybrid. RF LO. IF 90º Hybrid. A. IF1 IF2. B. LPF. ID2'. HPF. ID2. (a). VRF,A. VLO,A. ID1,RF. ID1,LO. VRF,B. VLO,B. ID2,RF. ID2.LO. (b). Figure 3.10: (a) Topology of UWB subharmonic IRM (b) Diode current configuration I. VLO(t) gLO(t). 54 t. V. 1/f.
(73) 0 After ID1 and ID2 going through the IF directional coupler, outputs IF1 and IF2. can be expressed as. 0 IF 1 = ID1 + (−j)ID2 = ID1 + (j)m+1 (−j)n ID1 = (1 + (j)m+1 (−j)n )ID1. (3.10). 0 IF 2 = −jID1 + ID2 = −jID1 − (j)m (−j)n ID1 = −(j + (j)m (−j)n )ID1. (3.11). We now see what signal will going out when harmonic index when (m,n) equals (1,-2). IF 1 = (1 + (j)2 (−j)−2 )ID1 = (1 + (−1)(−1))ID1 = 2ID1. (3.12). IF 2 = −(j + (j)1 (−j)−2 )ID1 = −(j + j(−1))ID1 = 0. (3.13). this means that no signal component will show on IF2 when fIF = fRF − 2fLO . When (m,n) equals (-1,2). IF 1 = (1 + (j)0 (−j)2 )ID1 = (1 + 1(−1))ID1 = 0. (3.14). IF 2 = −(j + (j)−1 (−j)2 )ID1 = −(j + (−j)(−1))ID1 = −2jID1. (3.15). 2fLO − fRF only appears on IF2. If the wanted signal is fIF = fRF − 2fLO , then 2fLO − fRF is the image signal. Therefore, the output signal will appear on IF1 and the image signal is totally eliminated by this proposed subharmonic IRM. As the same results, if fIF = 2fLO − fRF is the wanted signal, the image signal is eliminated and the output signal will appear on IF2. The fundamental mixing signal appears when (m,n) equals (1,-1) or (-1,1). For 55.
(74) (m,n) equals (1,-1) IF 1 = (1 + (j)2 (−j)−1 )ID1 = (1 + (−1)j)ID1 = (1 − j)ID1. (3.16). IF 2 = −(j + (j)1 (−j)−1 )ID1 = −(j + j(j))ID1 = (1 − j)ID1. (3.17). and (m,n) equals (-1,1) IF 1 = (1 + (j)0 (−j)1 )ID1 = (1 − j)ID1. (3.18). IF 2 = −(j + (j)−1 (−j)1 )ID1 = −(j + (−j)(−j))ID1 = (1 − j)ID1. (3.19). The above two equations show that not only the fundamental mixing signal but also the image signal reach output ports.. 3.4.2. Circuit Realization and Measurements. Photograph and configuration of the proposed UWB subharmonic image rejection mixer are shown in Fig. 3.11 and Fig. 3.12. MA-COM JHS-115, which is a surface mount quadrature hybrid with bandwidth of 40-80MHz, is used as the IF quadrature hybrid of the proposed mixer. The total circuit consists of a UWB RF/LO directional coupler, two diodes, a low-pass filter, a high-pass filer, and an IF quadrature hybrid. Fig. 3.13 shows the conversion loss versus local power of the image rejection mixer. The conversion loss is lower than 15dB when local power is larger than 8dBm. Fig. 3.14 is the graph of RF to IF conversion loss versus RF frequency, conversion loss of image signal is also shown in this figure. The conversion loss of RF to IF is lower than 20dB when RF frequency is from 3-13GHz, totally covered the entire UWB bandwidth. 56.
(75) Figure 3.11: Photograph of the proposed UWB subharmonic IRM. 57.
(76) 3.13. Conversion L. LO. 20. 15. 10 0. 20. 40. RF. 60. 80. 100. 120. IF. IF Frequency (MHz). Bond wire. 25. Image Rejection (dB). 20. 15. 10. IF hybrid. 5. IF. LO 0 0. 20. 40. 60. 80. 100. 120. Figure 3.12: UWB subharmonic IRM circuit configuration IF Frequency (MHz) 45. 40. Conversion Loss (dB). 3.16 3.17. IF. 25. 35. 30. 25. 20. 15. 10 0. 2. 4. 6. 8. 10. 12. 14. LO Power (dBm). Figure 3.13: Conversion loss versus local power of IRM 58. 16.
(77) 45. IF Image. 40. Conversion Loss (dB). Col 1 vs Col 17 35. 30. 25. 20. 15. Mean conversion loss = 16.5dB 10 2. 4. 6. 8. 10. 12. 14. RF Frequency (GHz). Figure 3.14: Conversion loss versus RF frequency of IRM Image rejection ratio is shown in Fig. 3.15. Most of the frequencies in UWB 25. bandwidth have the image rejection ration larger than 15dB, which is a basic spec20. Image rejection (dB). ification of a wideband image rejection mixer. Fig. 3.16 shows the IF bandwidth of the mixer. Conversion loss is measured 15. under the following conditions. LO frequency is fixed at 4GHz and varying RF 10. frequency from 8.01GHz to 8.11GHz. The mixer can have a better performance from low IF frequency of about 40MHz to 80MHz. Fig. 3.17 is the image rejection 5. ratio versus IF frequency and Fig. 3.18 is the isolation of LO to RF versus RF frequency.. 0 2. 4. 6. 8. RF frequency (GHz). 59 3.14 3.15. 10. 12. 14.
(78) 15. 10 2. 4. 6. 8. 10. 12. 14. 10. 12. 14. RF Frequency (GHz). 25. Image rejection (dB). 20. 15. 10. 5. 0 2. 4. 6. 8. RF frequency (GHz). Figure 3.15: Image rejection ratio versus RF frequency of IRM. 45. Conversion Loss (dB). 40. IF Image. 3.14 3.15. 35. 30. 25. 20. 15. 10 0. 20. 40. 60. 80. 100. IF Frequency (MHz). Figure 3.16: Conversion loss versus IF frequency of IRM 25. 3.16 3.17. ection (dB). 20. 15. 60. 120.
(79) Co. 20. 15. 10 0. 20. 40. 60. 80. 100. 120. 100. 120. IF Frequency (MHz). 25. 3.16 3.17. (dB) ConversionImage Loss Rejection (dB). 40 20. 35 15 30. 10 25. 5 20. 0 150. 20. 40. 60. 80. IF Frequency (MHz) 10 0. 2. 4. 6. 8. 10. 12. 10. 12. 14. 16. Figure 3.17: Image rejection ratio versus IF frequency of IRM Local Power (dBm). 45. 3.13. Conversion Isolation (dB) Loss (dB). 40 26 35 24 30 22 25 20 20 18 15 16 10 14. 0. 2. 4. 6. 8. 14. 16. LO Power (dBm). 12 2. 4. 6. 8. 10. 12. 14. RF Frequency (GHz). Figure 3.18: Isolation of LO to RF versus RF frequency of IRM. 61.
(80) 62.
(81) Chapter 4 Conclusion. 63.
(82) In this thesis, a five-section 3-dB quadrature hybrid realized by conventional PCB process with Rogers RO4003 has been successfully demonstrated. The proposed modified VIP couplers have solved all three of the problems that a multisection 3-dB quadrature hybrid has always encountered, including an extremely tight-coupled center section, equalizing modal phase velocities, and minimizing the discontinuity effect between each section. VIP couplers with compensated stubs have shown that return loss and isolation can be effectively improved. The proposed directional coupler has shown a near-constant coupling over an ultra-wideband frequency bandwidth of 1.5-13.5GHz, return loss and isolation are better than 13dB over the entire bandwidth. We have demonstrated an UWB subharmonic quadrature-IF mixer with conversion loss better than 15dB when RF frequency is from 3-13GHz. The minimum layout area and fewest numbers of elements are the benefits of this mixer. I/Q amplitude deviation has shown fewer than 3dB in the bandwidth. An UWB subharmonic image rejection mixer has also demonstrated in this thesis. The mixer, when connected with an IF directional coupler, exhibits 1518dB conversion loss and 10-22dB of image rejection. IF bandwidth can cover the frequency range about 40-80MHz due to the limitation of the IF directional coupler.. 64.
(83) Bibliography [1] V. Sedghi, A. Abdipour, and A. Mohammadi, ”A flat conversion gain subharmonically pumped image rejection mixer in mm-wave band” Microwave and Millimeter Wave Technology, ICMMT 4th International Conference Aug. 2004, pp. 312 - 315. [2] P. Blount, ”An LMDS subharmonically pumped image reject mixer” GaAs IC Symposium, 23rd Annual Technical Dig., pp. 41 - 44, Oct. 2001. [3] E. G. Cristal, and L. Young, ”Theory and tTables of optimum symmetrical TEM-mode coupled transmission line directional couplers,” IEEE Trans. Microw. Theory Tech., vol. MTT-13, pp. 544-558, Sept. 1965. [4] S. Uystal and H. Aghvami, ”Synthesis,design, and construction of ultra-wideband nonuniform quadrature directional couplers in inhomogeneous media,” IEEE Trans. Microw. Theory Tech., vol. MTT-37, no. 6, pp. 969-976, Jun. 1989. [5] Duncan K. Y. Lau, Steve P. Marsh, L. E. Davis, and Robin Sloan, ”Simplified design technique for high-performance microstrip multisection couplers,” IEEE Trans. Microw. Theory Tech., vol. MTT-46, no. 12, pp. 2507-2513, Dec. 1998. 65.
(84) [6] R. M. Osmani, ”Synthesis of Lange couplers,” IEEE Trans. Microw. Theory Tech., vol. MTT-29, no. 12, pp. 168-170, Feb. 1981. [7] E.M. Bastida and N. Fanelli, ”Interdigitated coplanar directional couplers,” Electron. Lett., vol. 16, no. 17, pp. 645-646, 1980. [8] A. M. Pavio, and S. K. Sutton, ”A microstrip re-entrant mode quadrature coupler for hybrid and monolithic circuit applications,” IEEE MTT-S Symp., Dig., pp. 573-576, May. 1990. [9] L. Lavendol, and J. J. Taub, ”Re-entrant directional coupler using strip transmission line,” IEEE Trans. Microw. Theory Tech., vol. MTT-13, pp. 700-701, Sep. 1965. [10] S. B. Cohn, ”The re-entrant cross section and wideband 3-dB hybrid couplers,” IEEE Trans. Microw. Theory Tech., vol. MTT-11, pp. 254-258, Jul. 1963. [11] Shelton et al., ”Tandem couplers and phase shifters for multi-octave bandwidth,” Microwave, vol. 4, pp. 14-19, Apr. 1965. [12] Y. Konishi, I. Awai, and Y. Fukuoka, ”Newly proposed vertically installed planar circuit and its application,” IEEE Trans. Broadcast., vol. BC-33, no. 1, pp. 1-7, Mar. 1987. [13] Y. Konishi, I. Awai, Y. Fukuoka, and M. Nakajima, ”A directional coupler of a vertically installed planar circuit structure,” IEEE Trans. Microw. Theory Tech., vol. MTT-36, no. 6, pp. 1057-1063, Jun. 1988. 66.
(85) [14] H.C. Chen and C.Y. Chang, ”Modified vertically installed planar couplers for ultrabroadband multisection quadrature hybrid,” IEEE Microwave and Wireless Components Letters, vol. 16, no. 8, pp. 446-448, Aug. 2006. [15] C. Zhao and I. Awai, ”Applications of the finite difference techniques to the compensated VIP 3dB directional coupler,” IEEE Trans. Microw. Theory Tech., vol. MTT-44, no. 11, pp. 2045-2052, Nov. 1996.. 67.
(86)
數據
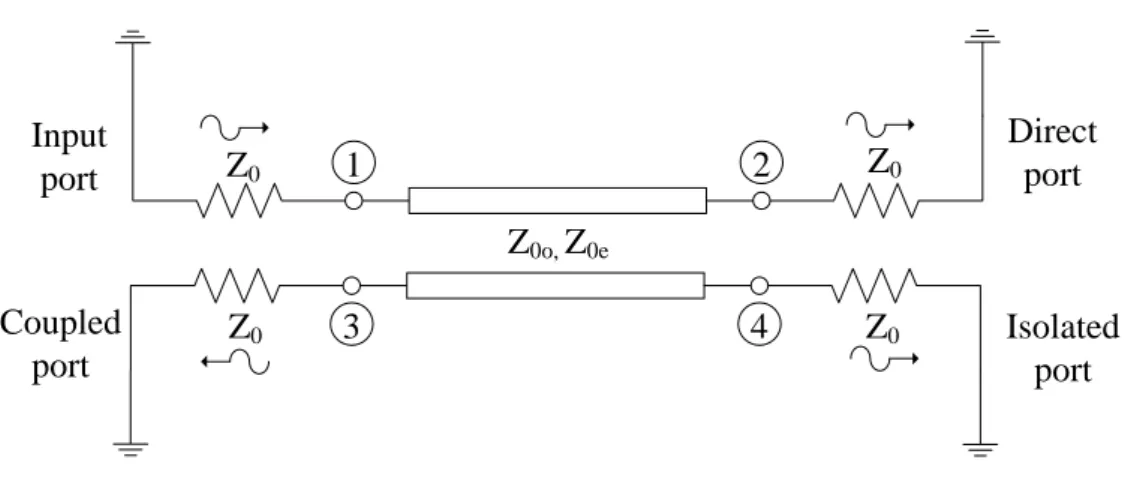
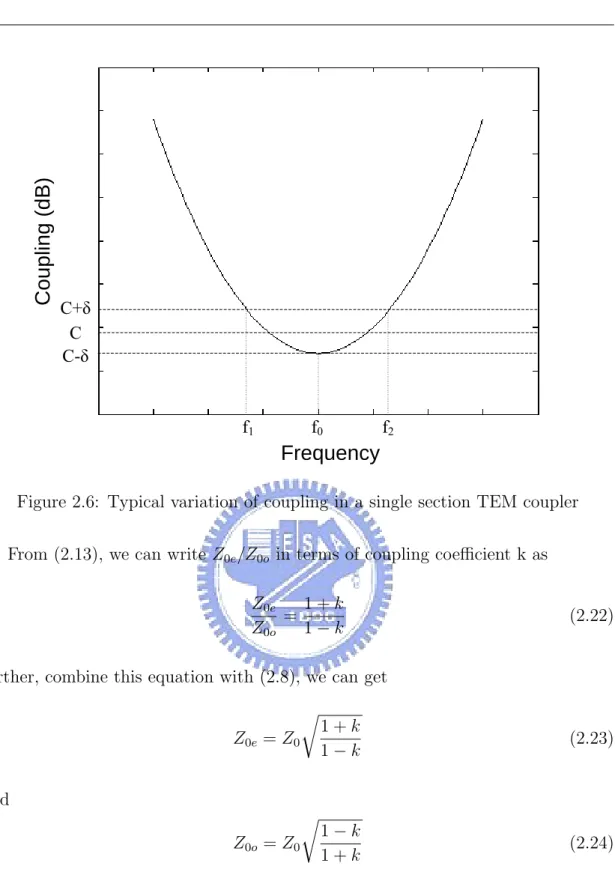
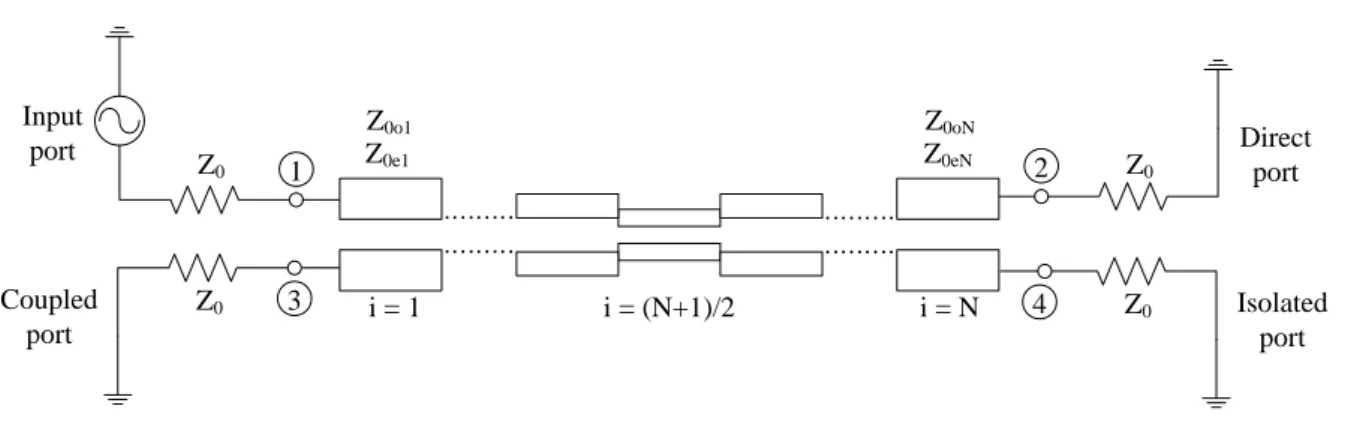
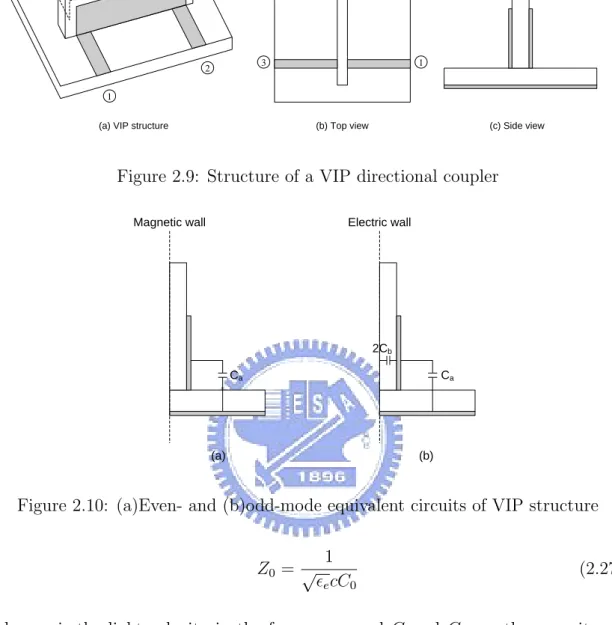
+7
相關文件
第四章 連續時間週期訊號之頻域分析-傅立葉級數 第五章 連續時間訊號之頻域分析-傅立葉轉換.. 第六章
Zhang, “Novel Microstrip Triangular Resonator Bandpass Filter with Transmission Zeros and Wide Bands Using Fractal-Shaped Defection,” Progress In Electromagnetics Research, PIER
定義為∣G(jω)∣降至零頻率增益(直流增益)值之 0.707 倍 時之頻率或-3dB 時頻率。.
了⼀一個方案,用以尋找滿足 Calabi 方程的空 間,這些空間現在通稱為 Calabi-Yau 空間。.
共集放大器 MATLAB 分析. CC
Promote project learning, mathematical modeling, and problem-based learning to strengthen the ability to integrate and apply knowledge and skills, and make. calculated
接收器: 目前敲擊回音法所採用的接收 器為一種寬頻的位移接收器 其與物體表
This kind of algorithm has also been a powerful tool for solving many other optimization problems, including symmetric cone complementarity problems [15, 16, 20–22], symmetric